Peter Rabl, Professor of Applied Quantum Theory at TUM and Scientific Director at WMI, has joined MCQST in 2023. His research includes hybrid quantum systems and quantum communication interfaces, modeling quantum systems for experimental use and developing new applications.
The bridge builder
Once you step into the researcher’s office, you can see right away that a theoretical physicist has moved in here. The whiteboard on the wall is already covered with formulas, operators and mathematical curves, and the first few books on quantum mechanics are already on the shelves. Peter Rabl, an Austrian physicist, moved into his office at the Walther-Meissner-Institute on the Garching campus over a year ago, and enjoys a view of a meadow and a stream. But despite all the indications, Peter Rabl is not a pure theoretician who is always sitting at his desk and scribbling equations that are difficult for the layperson to understand on a paper or on the whiteboard. "If you talked to a string theorist about my work, he would say I'm an experimental physicist," Rabl says and laughs.
And that is actually a bit true. Peter Rabl is a theorist, but his work is closely linked to its possible applications. As his title, Professor of Applied Quantum Theory, says, he hopes to use his calculations to help develop future quantum networks and make them more stable. Rabl is working on hybrid quantum systems and quantum communication interfaces. "I model quantum systems that other researchers use in the lab and develop new applications for these systems," explains Rabl.
Connecting quantum systems to one another
The researcher has moved to Munich in 2023. Before that, the 45-year-old did research in Innsbruck and most recently at the TU Wien. “During my studies in Innsbruck, a quantum physics lecture by Peter Zoller got me interested in theoretical physics and quantum optics," says Rabl. "That is what got me into quantum technologies." At the same time, he had always been fascinated by mathematical ideas. Rabl remained in Peter Zoller's department after graduating. There, he developed his most important basic idea as part of his doctoral thesis. Together with Zoller he became the first researcher to formulate the term "hybrid quantum processor" in 2006, which is the idea of combining different quantum systems that were being discussed at the time. Rabl was an early advocate of the modular principle, where you break down the operation of a quantum computer into its core tasks: computation, storage, and the distribution of information. He also worked closely with experimental physicists early on, for instance with Rainer Blatt from the University of Innsbruck, who is also on the Advisory Board of the MCQST Cluster of Excellence.
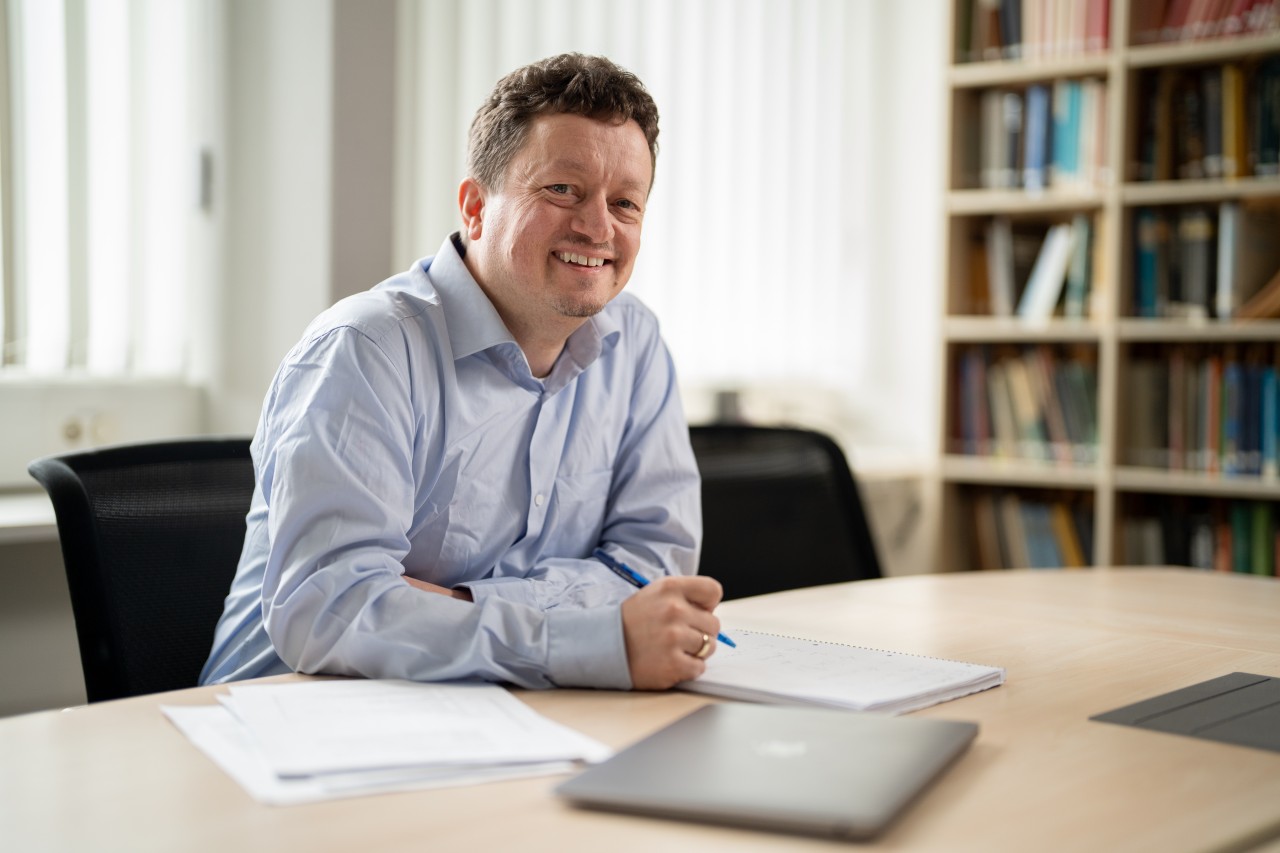
"If you talked to a string theorist about my work, he would say I'm an experimental physicist."
For a long time, the booming field of quantum technology, as Rabl explains, was starkly divided into different schools of thought. "Some wanted to build quantum computers with ions, others used superconducting circuits or worked with defects in diamonds, while still others experimented with electronic spins or photons." Each system has its advantages and disadvantages. "With superconducting circuits, for example, you can calculate quickly," explains Rabl. "But the quantum states don't survive very long, and the entanglement is quickly lost."
Entanglement is the word for the mysterious connection between distant quantum states. Entanglement is central to quantum computers because it is what enables calculations that are impossible for non-quantum computers. Their natural enemy is interfering fields from the environment, such as thermal radiation, which destroys the fragile quantum states. On the other hand, as Rabl explains in the interview, electron spins are very stable because they are influenced by their environment to a much lesser degree thanks to their weak magnetic interaction.
Battling the noise
It is a fascinating world that Rabl lays out, and you start to understand that, as a theorist, he wants to build bridges between systems, each of which has its own possibilities and advantages. And this is where he contributes his theoretical ideas. For instance, how quantum information can be transmitted reliably despite the disruptive noise in the connecting lines. Or, together with colleagues from Harvard, he proposes transmitting quantum information between two electrons with the help of phonons, the quanta of sound.
These are approaches so diverse that no experimental physicist could pursue them all. Rabl always tries to combine the theoretical advantages of the respective systems with the long-term objective of building better quantum computers, quantum simulators and networks overall. Calculations would be made with superconducting qubits – that much is clear, says Rabl. Quantum states would be stored in spin qubits and the exchange of information with other quantum computers would function with the help of photons using waveguides. Qubits are what researchers call the basic units of information in quantum computers. They possess quantum mechanical properties, such as entanglement.
Soon, there will be quantum computers with a thousand qubits
However, the quantum computers that have just been given so much attention are only at the beginning of their development. The basic elements of the computer architecture must first be developed piece by piece. However, momentum is picking up. High-tech companies like IBM or Google are reporting records for their quantum computers. IBM has introduced a device with 433 qubits, and there are rumours about the next largest system with 1,000 computing units. However, there is still a long way to go before we have powerful computers or even a quantum Internet. This would involve millions of qubits interacting with each other and lines between the computers that have to be cooled to temperatures just above absolute zero. Then there would be the matter of the fragility of the quantum systems, which continues to grow with size and must be virtually decoupled from the environment.
That is why quantum physicists like Peter Rabl all over the world are considering how to build systems that are more stable and, above all, larger. Scalability is a keyword here. Science predicts that if you have around 1,000 superconducting qubits in a single quantum computer, you will reach the limits of cooling. Although even larger cryogenic devices are imaginable, cooling is generally very challenging. Peter Rabl is also involved at a very special crossroads in this field; which has to do with the wiring of different computing units, so to speak.
Modular systems offer an answer
Rabl is also working with experimental physicists on this, in particular with German quantum physicist Andreas Wallraff from ETH Zurich, a top expert on superconducting connections between computing units. The researchers from Zurich have built cooled cables 30 meters long, a record. However, a lot of money is needed for this research, according to Rabl. And no one knows whether the principle will soon reach its limits. The EU is promoting these types of cooperation through the SuperQuLAN project, in which both Rabl and Wallraff are involved. The goal is to create large-scale local quantum networks (QuLANs) in which many individual superconducting quantum computer modules are interconnected modularly through coherent quantum communication channels.
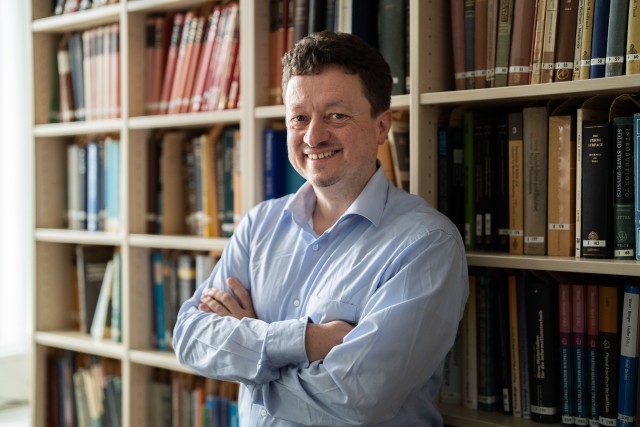
At the crossroads between theory and practice: “I want to make something tangible”
As a theoretician, Rabl can also pursue new concepts. He needs far less money for this than his colleagues in experimental physics. Rabl usually writes down his partial differential equations on a piece of paper or whiteboard and uses them to describe the system in question. “We understand in principle how the equations can be solved, but the number of parameters describing the system increases exponentially with the number of qubits," explains Rabl. Ten qubits can be simulated on every laptop, but twenty or thirty cannot, says Rabl. “The equations quickly become unsolvable.” So he attempts to simplify the systems of equations with the help of theoretical tools and physical considerations. "My job is to understand which parts of the system are important and which are negligible without losing important aspects of the physics." This means systems should start to become predictable for powerful computers. “I want to make something tangible that has some connection to reality,” explains Rabl. “To do that, you sometimes have to be a bit looser with the theory."
When talking with Peter Rabl, the topic often jumps back and forth a bit between equations and applications. For him, these seem to be one and the same. And so the theory and practical elements of his latest idea have also been developed together with experimental physicists from the Institute of Science and Technology Austria. For the first time, the team has entangled optical photons and microwave photons – it is a revolutionary approach. This is because it allows for superconducting, extremely cooled quantum circuits to be wired together via room temperature connections, meaning superconducting wires are no longer needed. It would be a huge step to link several smaller quantum units with a thousand qubits each to one another.
"Munich is unique with its Garching campus. There is a big push for quantum technologies here."
Instead of microwave photons, which are susceptible to noise and are necessary for calculations in the quantum computer, the researchers used optical photons with much higher frequencies similar to visible light. These are insensitive to temperature. The challenge was to make the microwave photons interact with the optical photons and entangle them. This path is appealing from a theoretical point of view, says Rabl. Practically, however, it does not yet work optimally. "But we were able to demonstrate that the approach could work. Now we have to improve the system.” And this is exactly where his models help, because they describe precisely how information could be transmitted. And parameters can be changed more quickly in models than in quantum experiments. It is apparent how much Rabl enjoys this role.
For these types of collaborations, the proximity between experimental physicists and theorists is a great advantage. "This is the only way to find the relevant questions," says Rabl. "That is the attraction of large international research projects. You always get new input.”
It was one of the reasons why he chose to come to Munich, to the Walther-Meissner-Institute, where he has the advantage of being close to many Munich institutions, to the MCQST Cluster of Excellence with its unique combination of different approaches, to Munich Quantum Valley, to the Max Planck Institutes. "Munich is unique with its Garching campus,” says Rabl. Many physicists are conducting research in similar areas, such as superconducting qubits. "There is a big push for quantum technologies here." He also sees this in the applications from young researchers who want to come to Munich. "The visibility is also high on an international scale thanks to the Munich cluster of excellence."
Related read
- Peter Rabl's research page
- Interview with Andreas Wallraf: Andreas Wallraff: "This perseverance is what distinguishes our group"