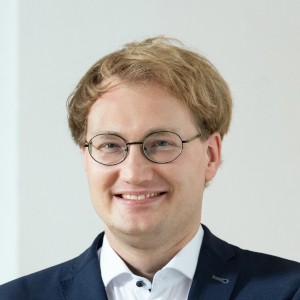
Technical University of Munich
TUM School of Computation, Information and Technology
Karlstr. 45-47
80333 Munich
+49 89 289 26902
tobias.vogl[at]tum.de
Research WebsiteDescription
Research focus: quantum cryptography, quantum theory, single photons, solid-state photonics, space systems
Our research is on optical quantum technologies using room temperature solid-state quantum systems. We are focusing on fluorescent defects in the 2D material hexagonal boron nitride that we combine with optical microcavities and photonic integrated circuits to build devices for quantum information applications. Our quantum devices are tested in quantum networks and satellite-based quantum communication links.
Quantum interferometry
Quantum mechanics is based on certain postulates, such as Born’s rule, which states that the probability density is given by the absolute square of the wavefunction. While it is impossible to prove this without making other assumptions on the mathematical structure of the measurement process, one can make interferometric experiments and check if the results follow the distribution predicted by Born’s rule. A consequence of any deviation would be higher-order interference in multi-path interferometers.
We have shown that a fundamental quantum advantage is possible when using true single photons instead of coherent states produced by a laser in interferometers. This allowed us to increase the sensitivity and find a tight upper bound to any potential deviation. Now we are increasing the phase
Our research is on optical quantum technologies using room temperature solid-state quantum systems. We are focusing on fluorescent defects in the 2D material hexagonal boron nitride that we combine with optical microcavities and photonic integrated circuits to build devices for quantum information applications. Our quantum devices are tested in quantum networks and satellite-based quantum communication links.
Quantum interferometry
Quantum mechanics is based on certain postulates, such as Born’s rule, which states that the probability density is given by the absolute square of the wavefunction. While it is impossible to prove this without making other assumptions on the mathematical structure of the measurement process, one can make interferometric experiments and check if the results follow the distribution predicted by Born’s rule. A consequence of any deviation would be higher-order interference in multi-path interferometers.
We have shown that a fundamental quantum advantage is possible when using true single photons instead of coherent states produced by a laser in interferometers. This allowed us to increase the sensitivity and find a tight upper bound to any potential deviation. Now we are increasing the phase stability of our interferometer and using more complex multi-path interferometers to further enhance the sensitivity of our experiments.
Quantum cryptography
The security of modern cryptographic systems used today is based on unproven mathematical assumptions that could be disproved at any time. Moreover, future quantum computers will be able to systematically break our public key cryptography. In contrast, quantum cryptography is based on fundamental laws of quantum mechanics: the no-cloning theorem, which states that no unknown quantum state can be copied perfectly and the Heisenberg uncertainty, which states that not all properties of a quantum system can be readout simultaneously. Encoding in single photons is crucial, as only then the information is protected from eavesdropping attacks.
Many implementations of quantum cryptography utilize weak coherent states. To reduce the amount of multi-photon pulses, a very low mean photon number is used, which implicates that most pulses are actually empty and carry no information. Instead, we are using single photons emitted from fluorescent defects in solid-state crystals. By using a true single photon source, we can enhance the data rate significantly. While our current experiments are still in a laboratory, in the near future we will also test free space links in the field and establish quantum links between distant buildings. In addition, we have developed a scheme where roof-to-roof or space-to-ground links can operate in ambient conditions, i.e., during daylight.
Single photon sources
Optical quantum technologies require sources of true photon sources. Their applications include quantum cryptography, fundamental quantum optics experiments, quantum computing, as well as metrology and sensing. A suitable process is the fluorescence of a single two-level quantum system because the excitation and subsequent decay to the ground state takes a finite time. The emitter can therefore only emit a single photon.
We use defects in the 2D material hexagonal boron nitride as a quantum light emitting platform. These emitters have a high quantum efficiency and short excited-state lifetime at room temperature, which results in a high single photon luminosity. Moreover, the 2D crystal lattice leads to near-ideal outcoupling, as emitters in atomically thin materials are not surrounded by any high refractive index material. At the moment we are combining our emitters with optical systems, including microcavities and integrated waveguide circuits.
Space systems
Quantum keys encoded in single photons cannot be easily transmitted over long distances. Due to the no-cloning theorem, it is impossible to copy and amplify the quantum signal. In fibers, the finite absorption and scattering in silica (even at telecom wavelengths) limits the maximum communication distance to a few hundreds of kilometers. Longer distances can be bridged with satellites that can distribute quantum keys with distant optical ground stations around the world.
To develop a satellite that is capable of generating single photon states in orbit, we have miniaturized our single photon source based on a quantum emitter on hexagonal boron nitride. Operating instruments in space environments, however, requires rigorous qualification, including thermal-vacuum cycling, irradiation, as well as mechanical shock and vibration tests. As our payload has passed these tests on the individual component level, we are now integrating the entire system on a 3U CubeSat with the satellite launch scheduled for 2024.
Theoretical solid-state physics
To get insight into the electronic structure of color centers or fluorescent defects in solid-state crystals, we work on first-principle calculations, e.g., density functional theory (DFT), numerical post-processing DFT, and other numerical simulations to reveal the comprehensive photophysical fingerprint of specific defects. With our in-house developed workflow for calculating these properties, we are collecting a database of defects suitable for quantum communication, quantum sensing, and quantum memories. In addition, we are also working on novel tailoring mechanisms, which can be applied in our own experiments to achieve specifically desired properties.