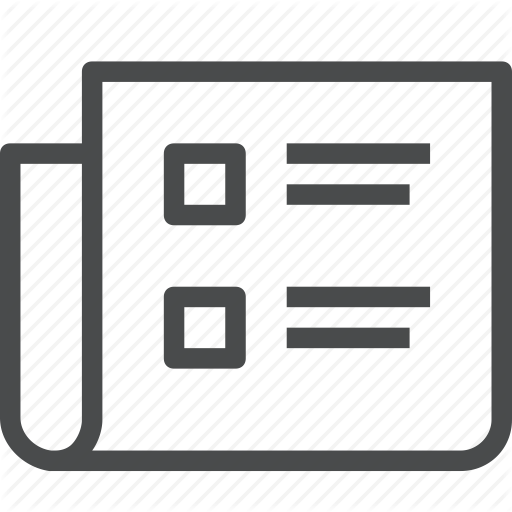
Real-space detection and manipulation of topological edge modes with ultracold atoms
C. Braun, R. Saint-Jalm, A. Hesse, J. Arceri, I. Bloch, M. Aidelsburger
Nature Physics 20 (2024).
The bulk-boundary correspondence, a fundamental principle relating the topological invariants of the bulk to the presence of edge states, is modified in periodically driven systems. Conventional bulk topological invariants are insufficient to predict the existence of topological edge modes in such systems. Although ultracold atoms provide excellent settings for clean realizations of Floquet protocols, the observation of real-space edge modes has so far remained elusive. Here we demonstrate an experimental protocol for realizing chiral edge modes in optical lattices through the periodic modulation of the tunnelling rate between neighbouring sites. In particular, we show how to efficiently prepare particles in edge modes in three distinct Floquet topological regimes in a periodically driven honeycomb lattice. Controlling the height and amplitude of the potential step, we characterize the emergence of edge modes and the dependence of their group velocity on the sharpness of the potential step. Our direct observation of topological edge modes provides a tool to study topological phases of matter in the presence of disorder and interactions, where conventional bulk observables are not applicable. The observation of edge modes in topological systems is challenging because precise control over the sample and occupied states is required. An experiment with atoms in a driven lattice now shows how edge modes with programmable potentials can be realized.
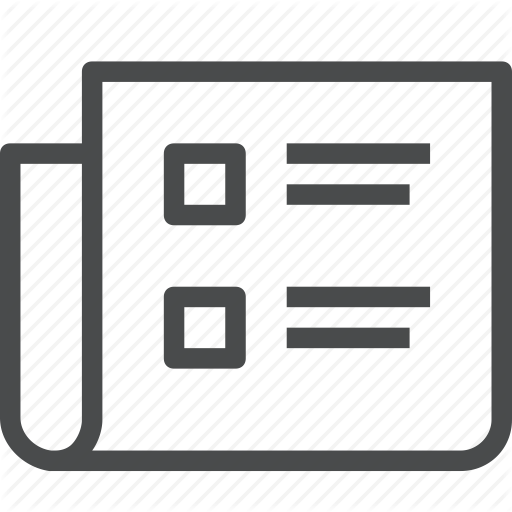
Fine-Structure Qubit Encoded in Metastable Strontium Trapped in an Optical Lattice
S. Pucher, V. Klüsener, F. Spriestersbach, J. Geiger, A. Schindewolf, I. Bloch, S. Blatt
Physical Review Letters 132 (15), 150605 (2024).
We demonstrate coherent control of the fine -structure qubit in neutral strontium atoms. This qubit is encoded in the metastable 3 P 2 and 3 P 0 states, coupled by a Raman transition. Using a magnetic quadrupole transition, we demonstrate coherent state initialization of this THz qubit. We show Rabi oscillations with more than 60 coherent cycles and single-qubit rotations on the mu s scale. With spin echo, we demonstrate coherence times of tens of ms. Our results pave the way for fast quantum information processors and highly tunable quantum simulators with two -electron atoms.
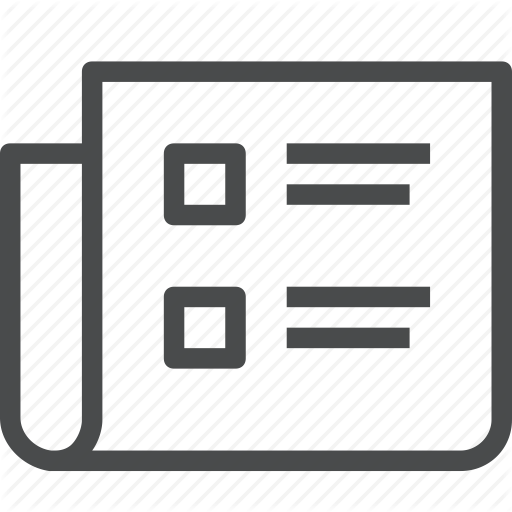
Equation of State and Thermometry of the 2D SU(N) Fermi-Hubbard Model
G. Pasqualetti, O. Bettermann, N. D. Oppong, E. Ibarra-García-Padilla, S. Dasgupta, R. T. Scalettar, K. R. A. Hazzard, I. Bloch, S. Fölling
Physical Review Letters 132 (8), 83401 (2024).
We characterize the equation of state (EoS) of the SU(N > 2) Fermi -Hubbard Model (FHM) in a twodimensional single -layer square optical lattice. We probe the density and the site occupation probabilities as functions of interaction strength and temperature for N = 3, 4, and 6. Our measurements are used as a benchmark for state-of-the-art numerical methods including determinantal quantum Monte Carlo and numerical linked cluster expansion. By probing the density fluctuations, we compare temperatures determined in a model -independent way by fitting measurements to numerically calculated EoS results, making this a particularly interesting new step in the exploration and characterization of the SU(N) FHM.
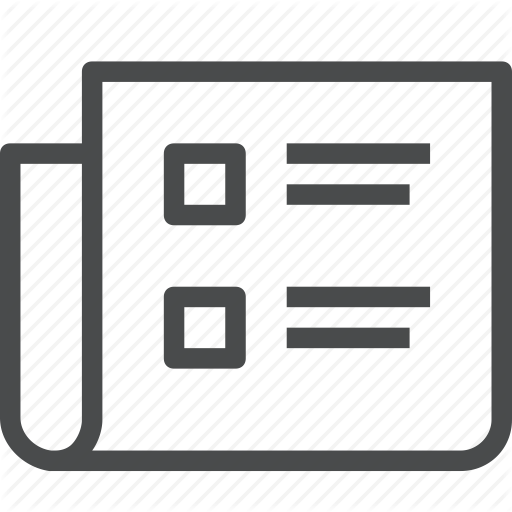
Ultracold field-linked tetratomic molecules
X. Y. Chen, S. Biswas, S. Eppelt, A. Schindewolf, F. L. Deng, T. Shi, S. Yi, T. A. Hilker, I. Bloch, X. Y. Luo
Nature 626 (7998), 15 (2024).
Ultracold polyatomic molecules offer opportunities1 in cold chemistry2,3, precision measurements4 and quantum information processing5,6, because of their rich internal structure. However, their increased complexity compared with diatomic molecules presents a challenge in using conventional cooling techniques. Here we demonstrate an approach to create weakly bound ultracold polyatomic molecules by electroassociation7 (F.D. et al., manuscript in preparation) in a degenerate Fermi gas of microwave-dressed polar molecules through a field-linked resonance8-11. Starting from ground-state NaK molecules, we create around 1.1 x 103 weakly bound tetratomic (NaK)2 molecules, with a phase space density of 0.040(3) at a temperature of 134(3) nK, more than 3,000 times colder than previously realized tetratomic molecules12. We observe a maximum tetramer lifetime of 8(2) ms in free space without a notable change in the presence of an optical dipole trap, indicating that these tetramers are collisionally stable. Moreover, we directly image the dissociated tetramers through microwave-field modulation to probe the anisotropy of their wavefunction in momentum space. Our result demonstrates a universal tool for assembling weakly bound ultracold polyatomic molecules from smaller polar molecules, which is a crucial step towards Bose-Einstein condensation of polyatomic molecules and towards a new crossover from a dipolar Bardeen-Cooper-Schrieffer superfluid13-15 to a Bose-Einstein condensation of tetramers. Moreover, the long-lived field-linked state provides an ideal starting point for deterministic optical transfer to deeply bound tetramer states16-18. Ultracold polyatomic molecules can be created by electroassociation in a degenerate Fermi gas of microwave-dressed polar molecules through a field-linked resonance.
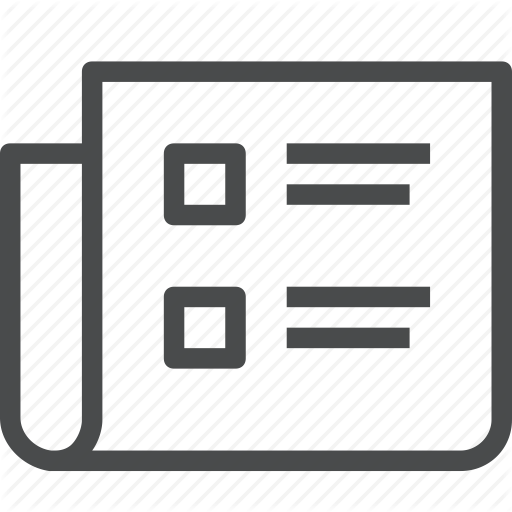
Transition from a polaronic condensate to a degenerate Fermi gas of heteronuclear molecules
M. Duda, X. Y. Chen, A. Schindewolf, R. Bause, J. von Milczewski, R. Schmidt, I. Bloch, X. Y. Luo
Nature Physics 19 (5), 17 (2023).
The interplay of quantum statistics and interactions in atomic Bose-Fermi mixtures leads to a phase diagram markedly different from pure fermionic or bosonic systems. However, investigating this phase diagram remains challenging when bosons condense due to the resulting fast interspecies loss. Here we report observations consistent with a phase transition from a polaronic to a molecular phase in a density-matched degenerate Bose-Fermi mixture. The condensate fraction, representing the order parameter of the transition, is depleted by interactions, and the build-up of strong correlations results in the emergence of a molecular Fermi gas. The features of the underlying quantum phase transition represent a new phenomenon complementary to the paradigmatic Bose-Einstein condensate/Bardeen-Cooper-Schrieffer crossover observed in Fermi systems. By driving the system through the transition, we produce a sample of sodium-potassium molecules exhibiting a large molecule-frame dipole moment in the quantum-degenerate regime. Tuning interspecies interactions in atomic Bose-Fermi mixtures is shown to drive the system through a quantum phase transition. This enables the generation of heteronuclear molecules in the quantum-degenerate regime.
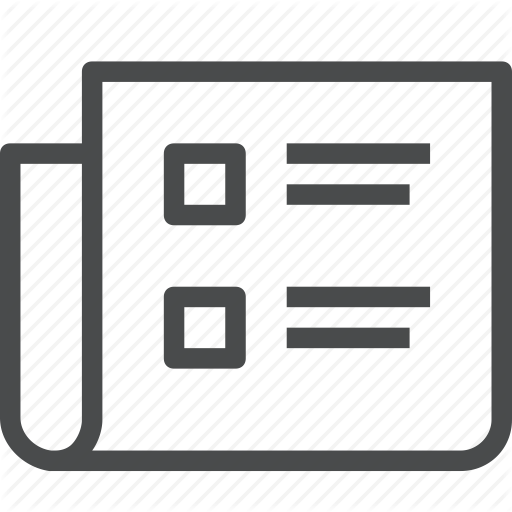
Clock-line photoassociation of strongly bound dimers in a magic-wavelength lattice
O. Bettermann, N. D. Oppong, G. Pasqualetti, L. Riegger, I. Bloch, S. Fölling
Physical Review A 108 (4), L041302 (2023).
We report on the direct optical production and spectroscopy of 1S0-3P0 molecules with large binding energy using the clock transition of 171Yb, and on the observation of the associated orbital Feshbach resonance near 1300 G. We measure the magnetic field dependence of the closed-channel dimer and of the open-channel pair state energy via clock-line spectroscopy in a deep optical lattice. In addition, we show that the free-to-bound transition into the dimer can be made first-order insensitive to the trap depth by the choice of lattice wavelength. Finally, we determine the fundamental intra- and interorbital scattering lengths and probe the stability of the corresponding pair states, finding long lifetimes in both interorbital interaction channels. These results are promising both for molecular clocks and for the preparation of strongly interacting multiorbital Fermi gases.
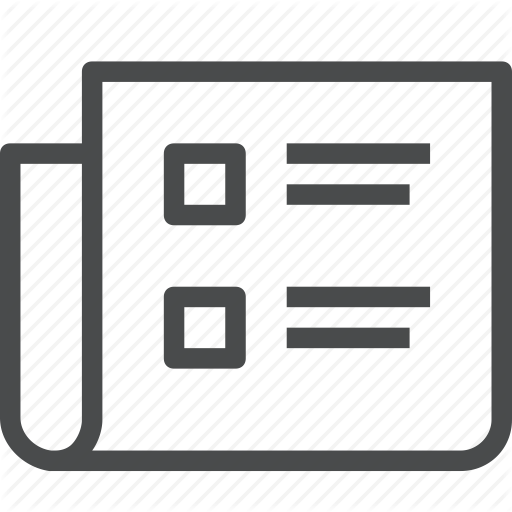
Quantifying hole-motion-induced frustration in doped antiferromagnets by Hamiltonian reconstruction
H. Schlömer, T. A. Hilker, I. Bloch, U. Schollwöck, F. Grusdt, A. Bohrdt
Communications Materials 4 (1), 64 (2023).
Unveiling the microscopic origins of quantum phases dominated by the interplay of spin and motional degrees of freedom constitutes one of the central challenges in strongly correlated many-body physics. When holes move through an antiferromagnetic spin background, they displace the positions of spins, which induces effective frustration in the magnetic environment. However, a concrete characterization of this effect in a quantum many-body system is still an unsolved problem. Here we present a Hamiltonian reconstruction scheme that allows for a precise quantification of hole-motion-induced frustration. We access non-local correlation functions through projective measurements of the many-body state, from which effective spin-Hamiltonians can be recovered after detaching the magnetic background from dominant charge fluctuations. The scheme is applied to systems of mixed dimensionality, where holes are restricted to move in one dimension, but SU(2) superexchange is two-dimensional. We demonstrate that hole motion drives the spin background into a highly frustrated regime, which can quantitatively be described by an effective J(1)-J(2)-type spin model. We exemplify the applicability of the reconstruction scheme to ultracold atom experiments by recovering effective spin-Hamiltonians of experimentally obtained 1D Fermi-Hubbard snapshots. Our method can be generalized to fully 2D systems, enabling promising microscopic perspectives on the doped Hubbard model.
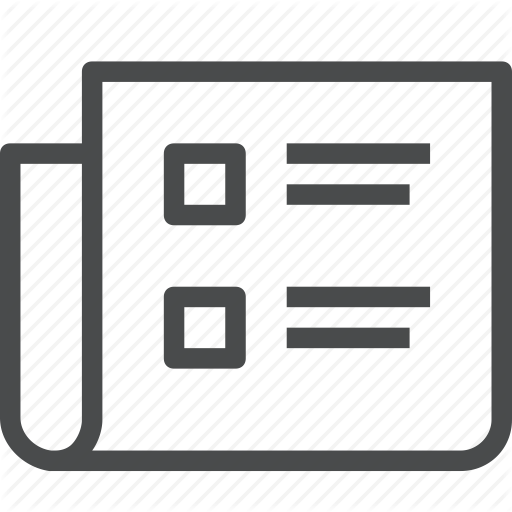
Preparing and Analyzing Solitons in the Sine-Gordon Model with Quantum Gas Microscopes
E. Wybo, A. Bastianello, M. Aidelsburger, I. Bloch, M. Knap
Prx Quantum 4 (3), 30308 (2023).
The sine-Gordon model emerges as a low-energy theory in a plethora of quantum many-body systems. Here, we theoretically investigate tunnel-coupled Bose-Hubbard chains with strong repulsive interactions as a realization of the sine-Gordon model deep in the quantum regime. We propose protocols for quantum gas microscopes of ultracold atoms to prepare and analyze solitons, which are the fundamental topological excitations of the emergent sine-Gordon theory. With numerical simulations based on matrix product states, we characterize the preparation and detection protocols and discuss the experimental requirements.
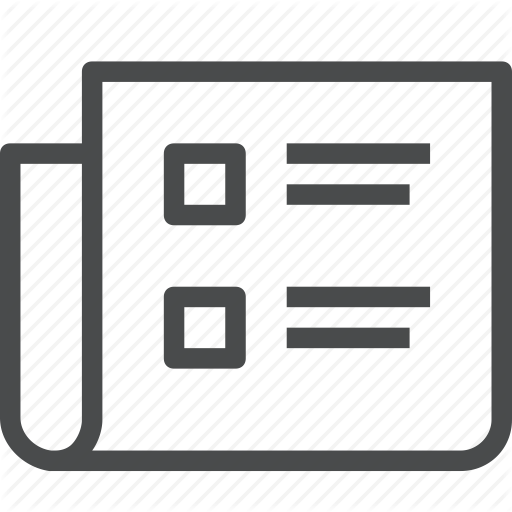
An unsupervised deep learning algorithm for single-site reconstruction in quantum gas microscopes
A. Impertro, J. F. Wienand, S. Häfele, H. von Raven, S. Hubele, T. Klostermann, C. R. Cabrera, I. Bloch, M. Aidelsburger
Communications Physics 6 (1), 166 (2023).
Quantum gas microscopy is an in-situ imaging technique used to investigate many-body phenomena in cold-atom quantum simulators and can provide resolution at the single-particle level,. however, limiting factors, such as short lattice constants and finite signal-to-noise ratios, weaken image resolution. Here, the authors develop an algorithm based on unsupervised deep learning that can reconstruct the occupation of an optical lattice of Cs atoms from fluorescence images with high fidelity. In quantum gas microscopy experiments, reconstructing the site-resolved lattice occupation with high fidelity is essential for the accurate extraction of physical observables. For short interatomic separations and limited signal-to-noise ratio, this task becomes increasingly challenging. Common methods rapidly decline in performance as the lattice spacing is decreased below half the imaging resolution. Here, we present an algorithm based on deep convolutional neural networks to reconstruct the site-resolved lattice occupation with high fidelity. The algorithm can be directly trained in an unsupervised fashion with experimental fluorescence images and allows for a fast reconstruction of large images containing several thousand lattice sites. We benchmark its performance using a quantum gas microscope with cesium atoms that utilizes short-spaced optical lattices with lattice constant 383.5 nm and a typical Rayleigh resolution of 850 nm. We obtain promising reconstruction fidelities ≳ 96% across all fillings based on a statistical analysis. We anticipate this algorithm to enable novel experiments with shorter lattice spacing, boost the readout fidelity and speed of lower-resolution imaging systems, and furthermore find application in related experiments such as trapped ions.
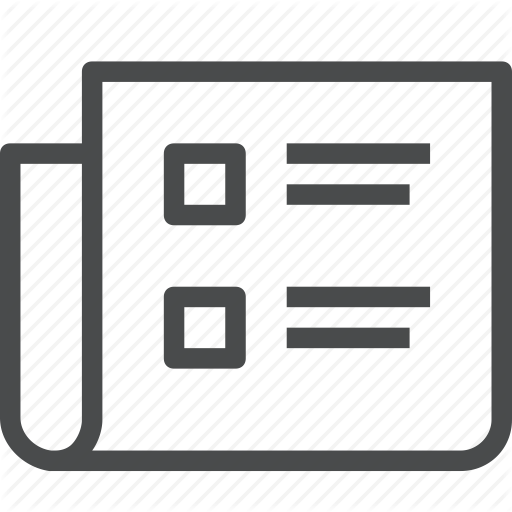
Observation of Brane Parity Order in Programmable Optical Lattices
D. Wei, D. Adler, K. Srakaew, S. Agrawal, P. Weckesser, I. Bloch, J. Zeiher
Physical Review X 13 (2), 21042 (2023).
The Mott-insulating phase of the two-dimensional (2D) Bose-Hubbard model is expected to be characterized by a nonlocal brane parity order. Parity order captures the presence of microscopic particle-hole fluctuations and entanglement, whose properties depend on the underlying lattice geometry. We realize 2D Bose-Hubbard models in dynamically tunable lattice geometries, using neutral atoms in a passively phase-stable tunable optical lattice in combination with programmable site-blocking potentials. We benchmark the performance of our system by single-particle quantum walks in the square, triangular, kagome, and Lieb lattices. In the strongly correlated regime, we microscopically characterize the geometry dependence of the quantum fluctuations and experimentally validate brane parity as a proxy for the nonlocal order parameter signaling the superfluid-to-Mott-insulating phase transition.
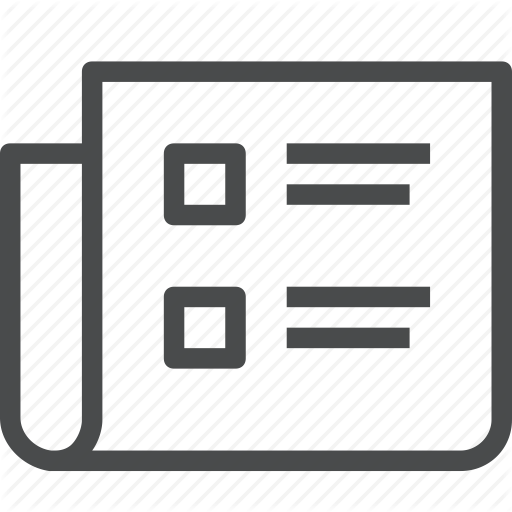
Long-lived fermionic Feshbach molecules with tunable p-wave interactions
M. Duda, X. Y. Chen, R. Bause, A. Schindewolf, I. Bloch, X. Y. Luo
Physical Review A 107 (5), 53322 (2023).
Ultracold fermionic Feshbach molecules are promising candidates for exploring quantum matter with strong p-wave interactions,. however, their lifetimes were measured to be short. Here we characterize the p-wave collisions of ultracold fermionic 23Na 40K Feshbach molecules for different scattering lengths and temperatures. By increasing the binding energy of the molecules, the two-body loss coefficient reduces by three orders of magnitude, leading to a second-long lifetime 20 times longer than that of ground-state NaK molecules. We exploit the scaling of elastic and inelastic collisions with the scattering length and temperature to identify a regime where the elastic collisions dominate over the inelastic ones, allowing the molecular sample to thermalize. Our results provide a benchmark for four-body calculations of molecular collisions and pave the way for investigating quantum many-body phenomena with fermionic Feshbach molecules.
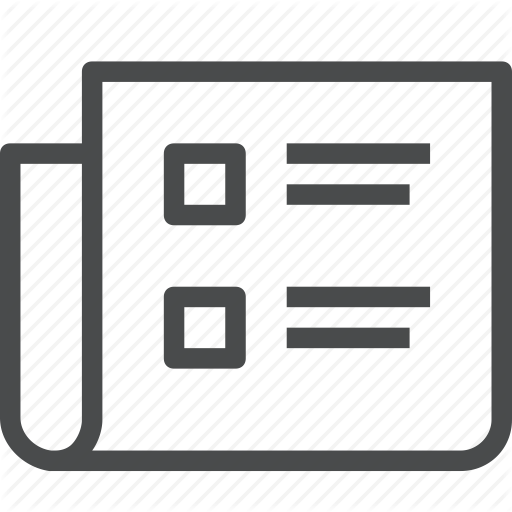
1S0-3P2 magnetic quadrupole transition in neutral strontium
J. Trautmann, D. Yankelev, V. Klüsener, A. J. Park, I. Bloch, S. Blatt
Physical Review Research 5 (1), 13219 (2023).
We present a detailed investigation of the ultranarrow magnetic-quadrupole S-1(0)-P-3(2) transition in neutral strontium and show how it can be made accessible for quantum simulation and quantum computation. By engineering the light shift in a one-dimensional optical lattice, we perform high-resolution spectroscopy and observe the characteristic absorption patterns for a magnetic quadrupole transition. We measure an absolute transition frequency of 446, 647, 242, 704(2) kHz in Sr-88 and an Sr-88-Sr-87 isotope shift of +62.91(4) MHz. In a proof-of-principle experiment, we use this transition to demonstrate local addressing in an optical lattice with 532 nm spacing with a Rayleigh-criterion resolution of 494(45) nm. Our results pave the way for applications of the magnetic quadrupole transition as an optical qubit and for single-site addressing in optical lattices.
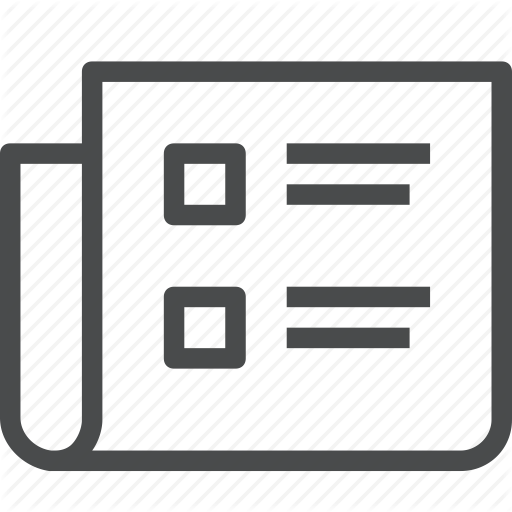
Field-linked resonances of polar molecules
X. Y. Chen, A. Schindewolf, S. Eppelt, R. Bause, M. Duda, S. Biswas, T. Karman, T. Hilker, I. Bloch, X. Y. Luo
Nature 614 (7946), 59-+ (2023).
Scattering resonances are an essential tool for controlling the interactions of ultracold atoms and molecules. However, conventional Feshbach scattering resonances(1), which have been extensively studied in various platforms(1-7), are not expected to exist in most ultracold polar molecules because of the fast loss that occurs when two molecules approach at a close distance(8-10). Here we demonstrate a new type of scattering resonance that is universal for a wide range of polar molecules. The so-called field-linked resonances(11-14) occur in the scattering of microwave-dressed molecules because of stable macroscopic tetramer states in the intermolecular potential. We identify two resonances between ultracold ground-state sodium-potassium molecules and use the microwave frequencies and polarizations to tune the inelastic collision rate by three orders of magnitude, from the unitary limit to well below the universal regime. The field-linked resonance provides a tuning knob to independently control the elastic contact interaction and the dipole-dipole interaction, which we observe as a modification in the thermalization rate. Our result provides a general strategy for resonant scattering between ultracold polar molecules, which paves the way for realizing dipolar superfluids(15) and molecular supersolids(16), as well as assembling ultracold polyatomic molecules.
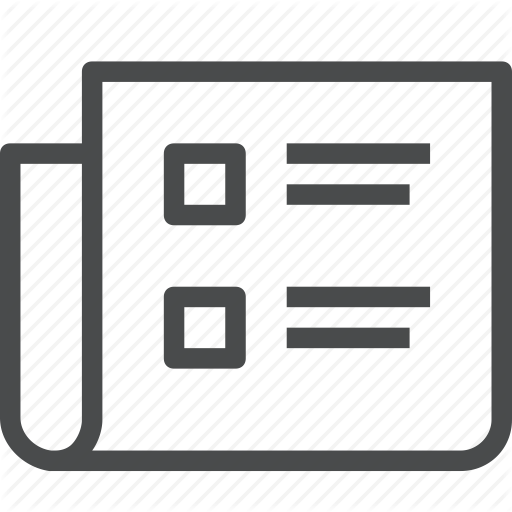
A subwavelength atomic array switched by a single Rydberg atom
K. Srakaew, P. Weckesser, S. Hollerith, D. Wei, D. Adler, I. Bloch, J. Zeiher
Nature Physics 19 (5), 7 (2023).
Enhancing light-matter coupling at the level of single quanta is essential for numerous applications in quantum science. The cooperative optical response of subwavelength atomic arrays has been found to open new pathways for such strong light-matter couplings, while simultaneously offering access to multiple spatial modes of the light field. Efficient single-mode free-space coupling to such arrays has been reported, but spatial control over the modes of outgoing light fields has remained elusive. Here, we demonstrate such spatial control over the optical response of an atomically thin mirror formed by a subwavelength array of atoms in free space using a single controlled ancilla atom excited to a Rydberg state. The switching behaviour is controlled by the admixture of a small Rydberg fraction to the atomic mirror, and consequently strong dipolar Rydberg interactions with the ancilla. Driving Rabi oscillations on the ancilla atom, we demonstrate coherent control of the transmission and reflection of the array. These results represent a step towards the realization of quantum coherent metasurfaces, the demonstration of controlled atom-photon entanglement and deterministic engineering of quantum states of light. The realization of efficient light-matter interfaces is important for many quantum technologies. An experiment now shows how to coherently switch the collective optical properties of an array of quantum emitters by driving a single ancilla atom to a Rydberg state.
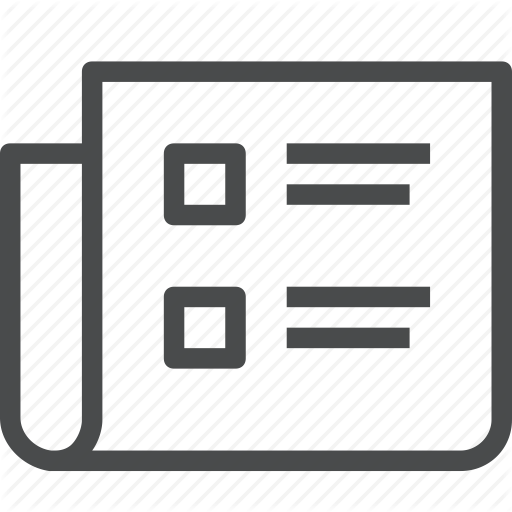
Ultracold Sticky Collisions: Theoretical and Experimental Status
R. Bause, A. Christianen, A. Schindewolf, I. Bloch, X. Y. Luo
Journal of Physical Chemistry A 13 (2023).
"Collisional complexes, which are formed as intermediate states in molecular collisions, are typically short-lived and decay within picoseconds. However, in ultracold collisions involving bialkali molecules, complexes can live for milliseconds, completely changing the collision dynamics. This can lead to unexpected two-body loss in samples of nonreactive molecules. During the past decade, such ""sticky"" collisions have been a major hindrance in the preparation of dense and stable molecular samples, especially in the quantum-degenerate regime. Currently, the behavior of the complexes is not fully understood. For example, in some cases, their lifetime has been measured to be many orders of magnitude longer than recent models predict. This is not only an intriguing problem in itself but also practically relevant, since understanding molecular complexes may help to mitigate their detrimental effects. Here, we review the recent experimental and theoretical progress in this field. We treat the case of molecule-molecule as well as molecule-atom collisions."
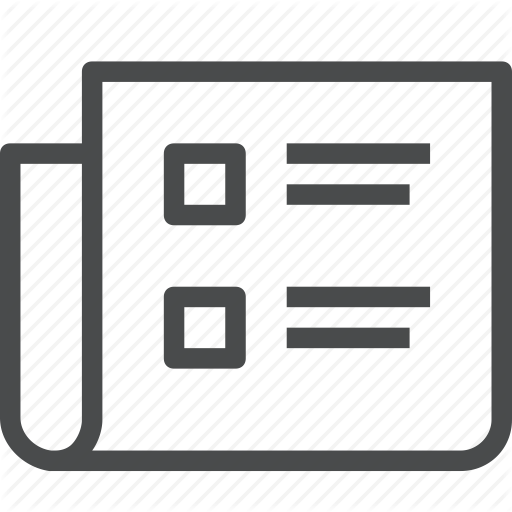
Magnetically mediated hole pairing in fermionic ladders of ultracold atoms
S. Hirthe, T. Chalopin, D. Bourgund, P. Bojovic, A. Bohrdt, E. Demler, F. Grusdt, I. Bloch, T. A. Hilker
Nature 613 (7944), 463-+ (2023).
Conventional superconductivity emerges from pairing of charge carriers-electrons or holes-mediated by phonons(1). In many unconventional superconductors, the pairing mechanism is conjectured to be mediated by magnetic correlations(2), as captured by models of mobile charges in doped antiferromagnets(3). However, a precise understanding of the underlying mechanism in real materials is still lacking and has been driving experimental and theoretical research for the past 40 years. Early theoretical studies predicted magnetic-mediated pairing of dopants in ladder systems(4-8), in which idealized theoretical toy models explained how pairing can emerge despite repulsive interactions(9). Here we experimentally observe this long-standing theoretical prediction, reporting hole pairing due to magnetic correlations in a quantum gas of ultracold atoms. By engineering doped antiferromagnetic ladders with mixed-dimensional couplings(10), we suppress Pauli blocking of holes at short length scales. This results in a marked increase in binding energy and decrease in pair size, enabling us to observe pairs of holes predominantly occupying the same rung of the ladder. We find a hole-hole binding energy of the order of the superexchange energy and, upon increased doping, we observe spatial structures in the pair distribution, indicating repulsion between bound hole pairs. By engineering a configuration in which binding is strongly enhanced, we delineate a strategy to increase the critical temperature for superconductivity.
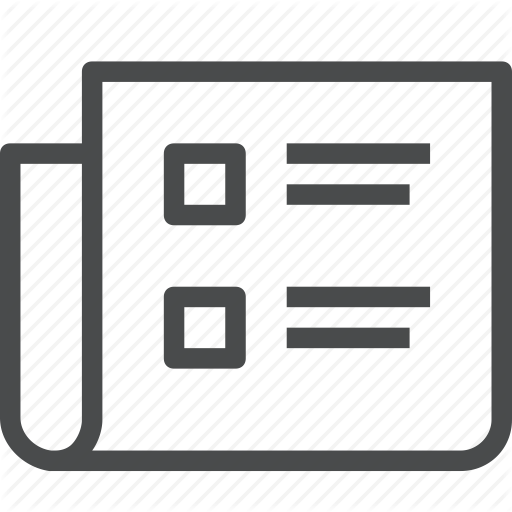
Exploring the Regime of Fragmentation in Strongly Tilted Fermi-Hubbard Chains
T. Kohlert, S. Scherg, P. Sala, F. Pollmann, B. H. Madhusudhana, I. Bloch, M. Aidelsburger
Physical Review Letters 130 (1), 10201 (2023).
Intriguingly, quantum many-body systems may defy thermalization even without disorder. One example is so-called fragmented models, where the many-body Hilbert space fragments into dynamically disconnected subspaces that are not determined by the global symmetries of the model. In this Letter we demonstrate that the tilted one-dimensional Fermi-Hubbard model naturally realizes distinct effective Hamiltonians that are expected to support nonergodic behavior due to fragmentation, even at resonances between the tilt energy and the Hubbard on site interaction. We find that the effective description captures the observed dynamics in experimentally accessible parameter ranges of moderate tilt values. Specifically, we observe a pronounced dependence of the relaxation dynamics on the initial doublon fraction, which directly reveals the microscopic processes of the fragmented model. Our results pave the way for future studies of nonergodic behavior in higher dimensions.
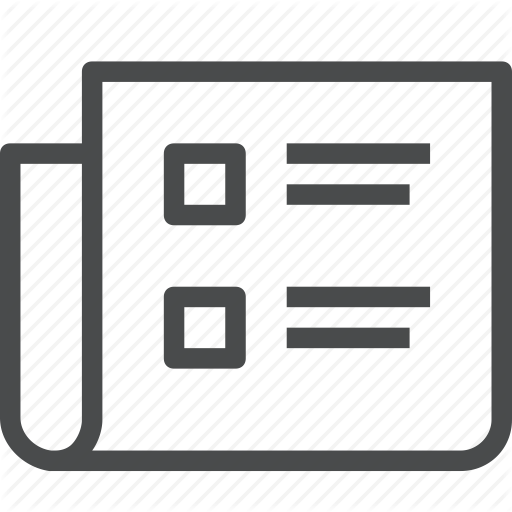
The superfluid-to-Mott insulator transition and the birth of experimental quantum simulation COMMENT
I. Bloch, M. Greiner
Nature Reviews Physics 4 (12), 739-740 (2022).
In 2002, an experiment with ultracold atoms emulated a textbook condensed-matter physics phenomenon: the phase transition from a superfluid to a Mott insulator. Two decades later, two of the researchers involved in that milestone experiment ponder how far quantum simulation with ultracold atoms has come.
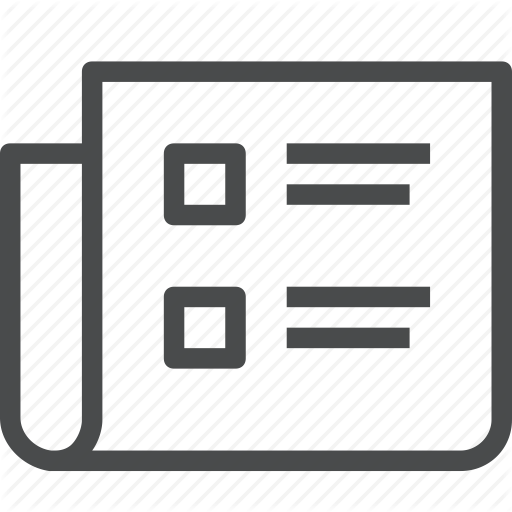
Propagation of errors and quantitative quantum simulation with quantum advantage
S. Flannigan, N. Pearson, G. Low, A. Buyskikh, I. Bloch, P. Zoller, M. Troyer, A. Daley
Quantum Science and Technology 7 (4), 45025 (2022).
The rapid development in hardware for quantum computing and simulation has led to much interest in problems where these devices can exceed the capabilities of existing classical computers and known methods. Approaching this for problems that go beyond testing the performance of a quantum device is an important step, and quantum simulation of many-body quench dynamics is one of the most promising candidates for early practical quantum advantage. We analyse the requirements for quantitatively reliable quantum simulation beyond the capabilities of existing classical methods for analogue quantum simulators with neutral atoms in optical lattices and trapped ions. Considering the primary sources of error in analogue devices and how they propagate after a quench in studies of the Hubbard or long-range transverse field Ising model, we identify the level of error expected in quantities we extract from experiments. We conclude for models that are directly implementable that regimes of practical quantum advantage are attained in current experiments with analogue simulators. We also identify the hardware requirements to reach the same level of accuracy with future fault-tolerant digital quantum simulation. Verification techniques are already available to test the assumptions we make here, and demonstrating these in experiments will be an important next step.
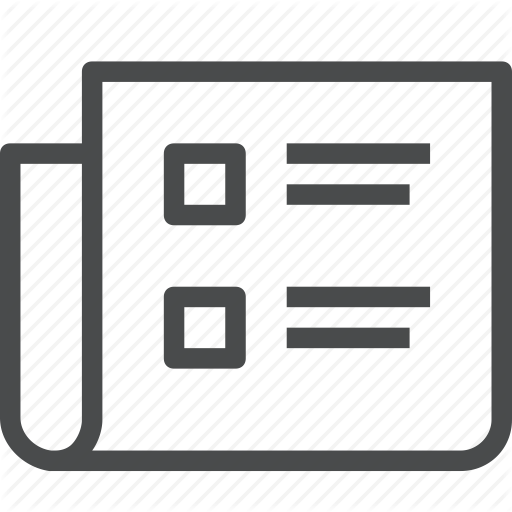
Probing Transport and Slow Relaxation in the Mass-Imbalanced Fermi-Hubbard Model
N. D. Oppong, G. Pasqualetti, O. Bettermann, P. Zechmann, M. Knap, I. Bloch, S. Folling
Physical Review X 12 (3), 31026 (2022).
Constraints in the dynamics of quantum many-body systems can dramatically alter transport properties and relaxation timescales even in the absence of static disorder. Here, we report on the observation of such constrained dynamics arising from the distinct mobility of two species in the one-dimensional mass-imbalanced Fermi-Hubbard model, realized with ultracold ytterbium atoms in a state-dependent optical lattice. By displacing the trap potential and monitoring the subsequent dynamical response of the system, we identify suppressed transport and slow relaxation with a strong dependence on the mass imbalance and interspecies interaction strength, consistent with eventual thermalization for long times. Our observations demonstrate the potential for quantum simulators to provide insights into unconventional relaxation dynamics arising from constraints.
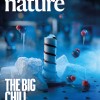
Evaporation of microwave-shielded polar molecules to quantum degeneracy
A. Schindewolf, R. Bause, X. Y. Chen, M. Duda, T. Karman, I. Bloch, X. Y. Luo
Nature 607 (7920), 677-+ (2022).
Ultracold polar molecules offer strong electric dipole moments and rich internal structure, which makes them ideal building blocks to explore exotic quantum matter(1-9), implement quantum information schemes(10-12) and test the fundamental symmetries of nature(13). Realizing their full potential requires cooling interacting molecular gases deeply into the quantum-degenerate regime. However, the intrinsically unstable collisions between molecules at short range have so far prevented direct cooling through elastic collisions to quantum degeneracy in three dimensions. Here we demonstrate evaporative cooling of a three-dimensional gas of fermionic sodium-potassium molecules to well below the Fermi temperature using microwave shielding. The molecules are protected from reaching short range with a repulsive barrier engineered by coupling rotational states with a blue-detuned circularly polarized microwave. The microwave dressing induces strong tunable dipolar interactions between the molecules, leading to high elastic collision rates that can exceed the inelastic ones by at least a factor of 460. This large elastic-to-inelastic collision ratio allows us to cool the molecular gas to 21 nanokelvin, corresponding to 0.36 times the Fermi temperature. Such cold and dense samples of polar molecules open the path to the exploration of many-body phenomena with strong dipolar interactions.
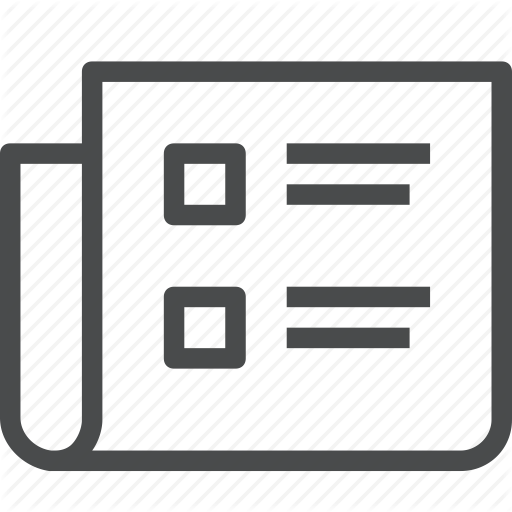
Cavity-Enhanced Optical Lattices for Scaling Neutral Atom Quantum Technologies to Higher Qubit Numbers
A. J. Park, J. Trautmann, N. Santic, V. Klusener, A. Heinz, I. Bloch, S. Blatt
Prx Quantum 3 (3), 30314 (2022).
We demonstrate a cavity-based solution to scale up experiments with ultracold atoms in optical lattices by an order of magnitude over state-of-the-art free-space lattices. Our two-dimensional (2D) optical lat-tices are created by power-enhancement cavities with large mode waists of 489(8) mu m and allow us to trap ultracold strontium atoms at a lattice depth of 60 mu K by using only 80 mW of input light per cavity axis. We characterize these lattices using high-resolution clock spectroscopy and resolve carrier transitions between different vibrational levels. With these spectral features, we locally measure the lattice potential envelope and the sample temperature with a spatial resolution limited only by the optical resolution of the imaging system. The measured ground-band and trap lifetimes are 18(3) s and 59(2) s, respectively, and the lattice frequency (depth) is long-term stable on the megahertz (0.1%) level. Our results show that large, deep, and stable 2D cavity-enhanced lattices can be created at any wavelength and can significantly increase the qubit number for neutral-atom-based quantum simulators, quantum computers, sensors, and optical-lattice clocks.
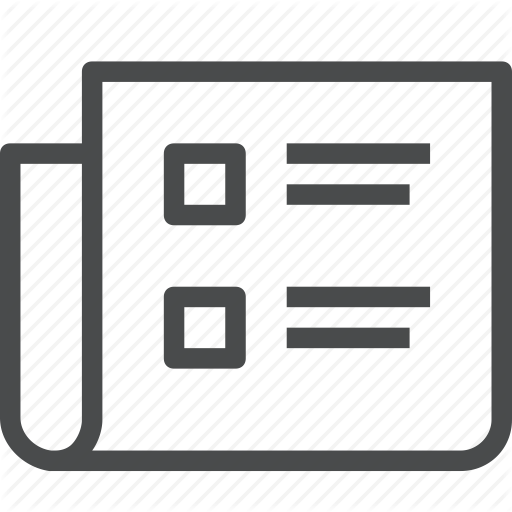
Practical quantum advantage in quantum simulation
A. J. Daley, I. Bloch, C. Kokail, S. Flannigan, N. Pearson, M. Troyer, P. Zoller
Nature 607 (7920), 667-676 (2022).
The development of quantum computing across several technologies and platforms has reached the point of having an advantage over classical computers for an artificial problem, a point known as 'quantum advantage'. As a next step along the development of this technology, it is now important to discuss 'practical quantum advantage', the point at which quantum devices will solve problems of practical interest that are not tractable for traditional supercomputers. Many of the most promising short-term applications of quantum computers fall under the umbrella of quantum simulation: modelling the quantum properties of microscopic particles that are directly relevant to modern materials science, high-energy physics and quantum chemistry. This would impact several important real-world applications, such as developing materials for batteries, industrial catalysis or nitrogen fixing. Much as aerodynamics can be studied either through simulations on a digital computer or in a wind tunnel, quantum simulation can be performed not only on future fault-tolerant digital quantum computers but also already today through special-purpose analogue quantum simulators. Here we overview the state of the art and future perspectives for quantum simulation, arguing that a first practical quantum advantage already exists in the case of specialized applications of analogue devices, and that fully digital devices open a full range of applications but require further development of fault-tolerant hardware. Hybrid digital-analogue devices that exist today already promise substantial flexibility in near-term applications.
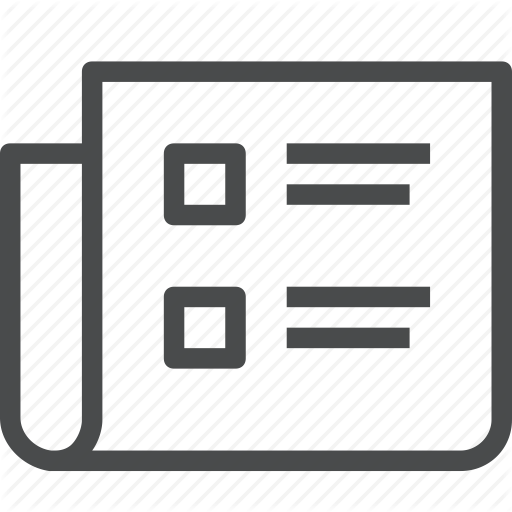
Realizing the symmetry-protected Haldane phase in Fermi-Hubbard ladders
P. Sompet, S. Hirthe, D. Bourgund, T. Chalopin, J. Bibo, J. Koepsell, P. Bojovic, R. Verresen, F. Pollmann, G. Salomon, C. Gross, T. A. Hilker, I. Bloch
Nature 606 (7914), 484-+ (2022).
Topology in quantum many-body systems has profoundly changed our understanding of quantum phases of matter. The model that has played an instrumental role in elucidating these effects is the antiferromagnetic spin-1 Haldane chain(1,2). Its ground state is a disordered state, with symmetry-protected fourfold-degenerate edge states due to fractional spin excitations. In the bulk, it is characterized by vanishing two-point spin correlations, gapped excitations and a characteristic non-local order parameter(3,4). More recently it has been understood that the Haldane chain forms a specific example of a more general classification scheme of symmetry-protected topological phases of matter, which is based on ideas connected to quantum information and entanglement(5-7). Here, we realize a finite-temperature version of such a topological Haldane phase with Fermi-Hubbard ladders in an ultracold-atom quantum simulator. We directly reveal both edge and bulk properties of the system through the use of single-site and particle-resolved measurements, as well as non-local correlation functions. Continuously changing the Hubbard interaction strength of the system enables us to investigate the robustness of the phase to charge (density) fluctuations far from the regime of the Heisenberg model, using a novel correlator.
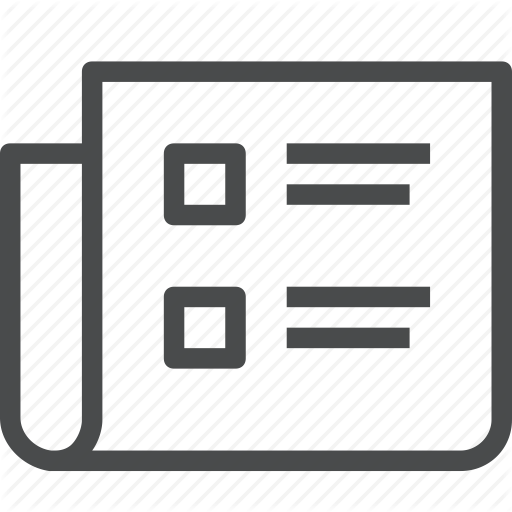
Strong pairing in mixed-dimensional bilayer antiferromagnetic Mott insulators
A. Bohrdt, L. Homeier, I. Bloch, E. Demler, F. Grusdt
Nature Physics 18 (6), 651-+ (2022).
Studies of unconventional pairing mechanisms in cold atoms require ultralow temperatures. Large-scale numerics show that certain bilayer models allow for deeply bound and highly mobile pairs of charges at more accessible temperatures. Interacting many-body systems in reduced-dimensional settings, such as ladders and few-layer systems, are characterized by enhanced quantum fluctuations. Recently, two-dimensional bilayer systems have sparked considerable interest because they can host unusual phases, including unconventional superconductivity. Here we present a theoretical proposal for realizing high-temperature pairing of fermions in a class of bilayer Hubbard models. We introduce a general and highly efficient pairing mechanism for mobile charge carriers in doped antiferromagnetic Mott insulators. The pairing is caused by the energy that one charge gains when it follows the path created by another charge. We show that this mechanism leads to the formation of highly mobile but tightly bound pairs in the case of mixed-dimensional Fermi-Hubbard bilayer systems. This setting is closely related to the Fermi-Hubbard model believed to capture the physics of copper oxides, and can be realized in currently available ultracold atom experiments.
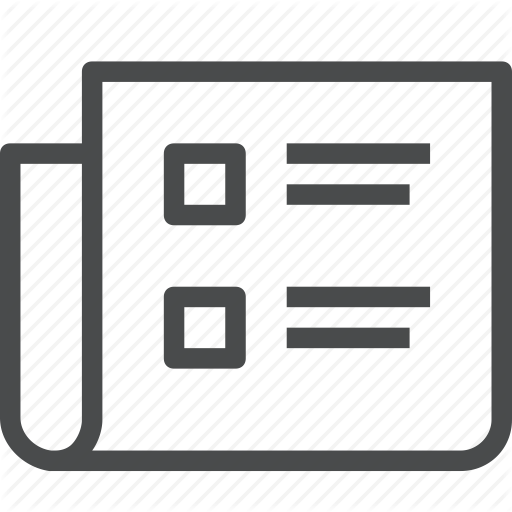
Quantum gas microscopy of Kardar-Parisi-Zhang superdiffusion
D. Wei, A. Rubio-Abadal, B. T. Ye, F. Machado, J. Kemp, K. Srakaew, S. Hollerith, J. Rui, S. Gopalakrishnan, N. Y. Yao, I. Bloch, J. Zeiher
Science 376 (6594), 716-+ (2022).
The Kardar-Parisi-Zhang (KPZ) universality class describes the coarse-grained behavior of a wealth of classical stochastic models. Surprisingly, KPZ universality was recently conjectured to also describe spin transport in the one-dimensional quantum Heisenberg model. We tested this conjecture by experimentally probing transport in a cold-atom quantum simulator via the relaxation of domain walls in spin chains of up to 50 spins. We found that domain-wall relaxation is indeed governed by the KPZ dynamical exponent z = 3/2 and that the occurrence of KPZ scaling requires both integrability and a nonabelian SU(2) symmetry. Finally, we leveraged the single-spin-sensitive detection enabled by the quantum gas microscope to measure an observable based on spin-transport statistics. Our results yield a clear signature of the nonlinearity that is a hallmark of KPZ universality.
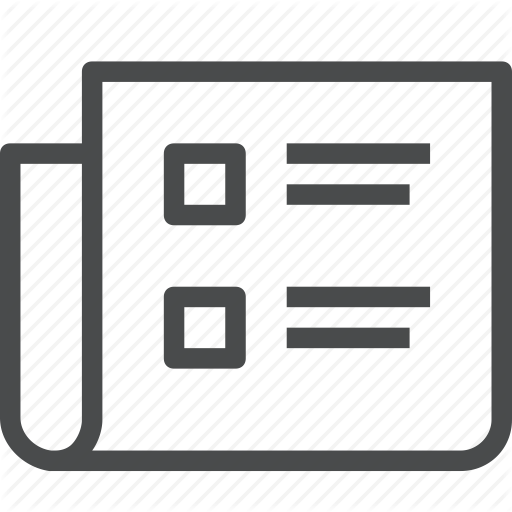
Fast long-distance transport of cold cesium atoms
T. Klostermann, C. R. Cabrera, H. von Raven, J. F. Wienand, C. Schweizer, I. Bloch, M. Aidelsburger
Physical Review A 105 (4), 43319 (2022).
Transporting cold atoms between distant sections of a vacuum system is a central ingredient in many quantum simulation experiments, in particular in setups, where large optical access and precise control over magnetic fields is needed. In this work, we demonstrate optical transport of cold cesium atoms over a total transfer distance of about 43 cm in less than 30 ms. The high speed is facilitated by a moving lattice, which is generated via the interference of a Bessel and a Gaussian laser beam. We transport about 3 x 10(6) atoms at a temperature of a few microkelvins with a transport efficiency of about 75%. We provide a detailed study of the transport efficiency for different accelerations and lattice depths and find that the transport efficiency is mainly limited by a fast initial loss most likely due to the sudden onset of the acceleration and the potential depth along the direction of gravity. To highlight the suitability of the optical-transport setup for quantum simulation experiments, we demonstrate the generation of a pure Bose-Einstein condensate with about 2 x 10(4) atoms. We find a robust final atom number within 2% over a duration of 2.5 h with a standard deviation of <5% between individual experimental realizations.
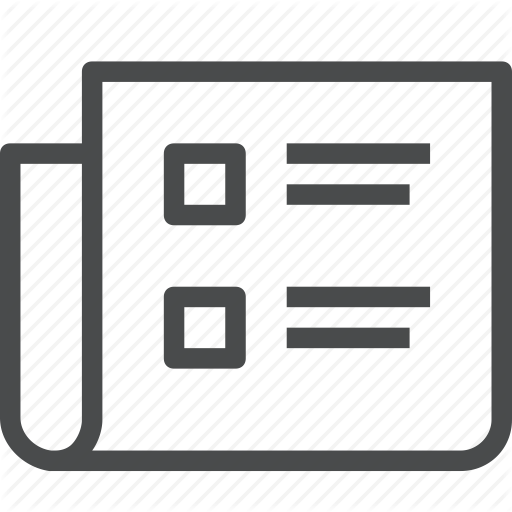
Suppression of Unitary Three-Body Loss in a Degenerate Bose-Fermi Mixture
X. Y. Chen, M. Duda, A. Schindewolf, R. Bause, I. Bloch, X. Y. Luo
Physical Review Letters 128 (15), 153401 (2022).
We study three-body loss in an ultracold mixture of a thermal Bose gas and a degenerate Fermi gas. We find that at unitarity, where the interspecies scattering length diverges, the usual inverse-square temperature scaling of the three-body loss found in nondegenerate systems is strongly modified and reduced with the increasing degeneracy of the Fermi gas. While the reduction of loss is qualitatively explained within the few-body scattering framework, a remaining suppression provides evidence for the long-range Ruderman-Kittel-Kasuya-Yosida (RKKY) interactions mediated by fermions between bosons. Our model based on RKKY interactions quantitatively reproduces the data without free parameters, and predicts one order of magnitude reduction of the three-body loss coefficient in the deeply Fermi-degenerate regime.
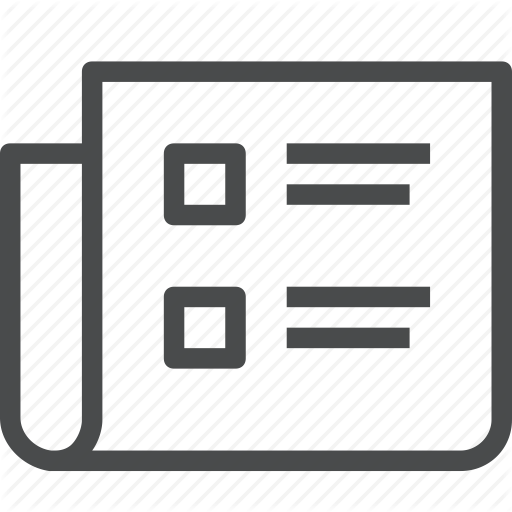
Realizing Distance-Selective Interactions in a Rydberg-Dressed Atom Array
S. Hollerith, K. Srakaew, D. Wei, A. Rubio-Abadal, D. Adler, P. Weckesser, A. Kruckenhauser, V. Walther, R. van Bijnen, J. Rui, C. Gross, I. Bloch, J. Zeiher
Physical Review Letters 128 (11), 113602 (2022).
Measurement-based quantum computing relies on the rapid creation of large-scale entanglement in a register of stable qubits. Atomic arrays are well suited to store quantum information, and entanglement can be created using highly-excited Rydberg states. Typically, isolating pairs during gate operation is difficult because Rydberg interactions feature long tails at large distances. Here, we engineer distance-selective interactions that are strongly peaked in distance through off-resonant laser coupling of molecular potentials between Rydberg atom pairs. Employing quantum gas microscopy, we verify the dressed interactions by observing correlated phase evolution using many-body Ramsey interferometry. We identify atom loss and coupling to continuum modes as a limitation of our present scheme and outline paths to mitigate these effects, paving the way towards the creation of large-scale entanglement.
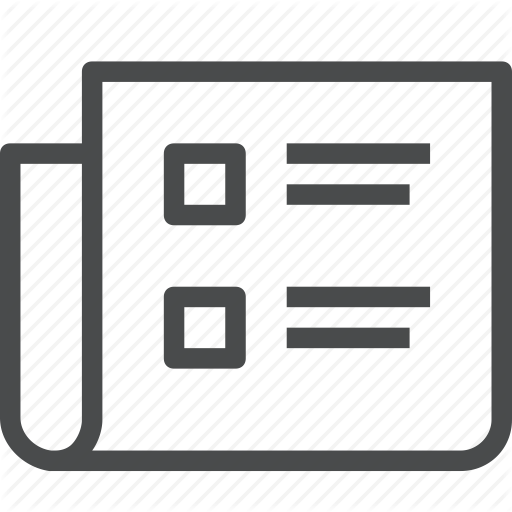
Benchmarking a Novel Efficient Numerical Method for Localized 1D Fermi-Hubbard Systems on a Quantum Simulator
B. H. Madhusudhana, S. Scherg, T. Kohlert, I. Bloch, M. Aidelsburger
Prx Quantum 2 (4), 40325 (2021).
Quantum simulators have made a remarkable progress towards exploring the dynamics of many-body systems, many of which offer a formidable challenge to both theoretical and numerical methods. While state-of-the-art quantum simulators are, in principle, able to simulate quantum dynamics well outside the domain of classical computers, they are noisy and limited in the variability of the initial state of the dynamics and the observables that can be measured. Despite these limitations, here we show that such a quantum simulator can be used to in effect solve for the dynamics of a many-body system. We develop an efficient numerical technique that facilitates classical simulations in regimes not accessible to exact calculations or other established numerical techniques. The method is based on approximations that are well suited to describe localized one-dimensional Fermi-Hubbard systems. Since this new method does not have an error estimate and the approximations do not hold in general, we use a neutral-atom Fermi-Hubbard quantum simulator with L-exp similar or equal to 290 lattice sites to benchmark its performance in terms of accuracy and convergence for evolution times up to 700 tunneling times. We then use these approximations in order to derive a simple prediction of the behavior of interacting Bloch oscillations for spin-imbalanced Fermi-Hubbard systems, which we show to be in quantitative agreement with experimental results. Finally, we demonstrate that the convergence of our method is the slowest when the entanglement depth developed in the many-body system we consider is neither too small nor too large. This represents a promising regime for near-term applications of quantum simulators.
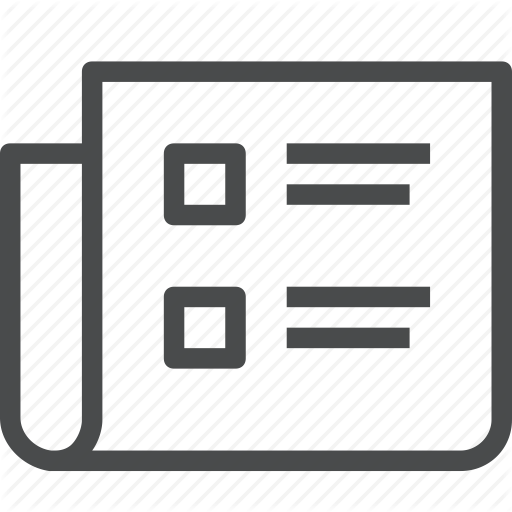
Microscopic evolution of doped Mott insulators from polaronic metal to Fermi liquid
J. Koepsell, D. Bourgund, P. Sompet, S. Hirthe, A. Bohrdt, Y. Wang, F. Grusdt, E. Demler, G. Salomon, C. Gross, I. Bloch
Science 374 (6563), 82-+ (2021).
The competition between antiferromagnetism and hole motion in two-dimensional Mott insulators lies at the heart of a doping-dependent transition from an anomalous metal to a conventional Fermi liquid. We observe such a crossover in Fermi-Hubbard systems on a cold-atom quantum simulator and reveal the transformation of multipoint correlations between spins and holes upon increasing doping at temperatures around the superexchange energy. Conventional observables, such as spin susceptibility, are furthermore computed from the microscopic snapshots of the system. Starting from a magnetic polaron regime, we find the system evolves into a Fermi liquid featuring incommensurate magnetic fluctuations and fundamentally altered correlations. The crossover is completed for hole dopings around 30%. Our work benchmarks theoretical approaches and discusses possible connections to lowertemperature phenomena.
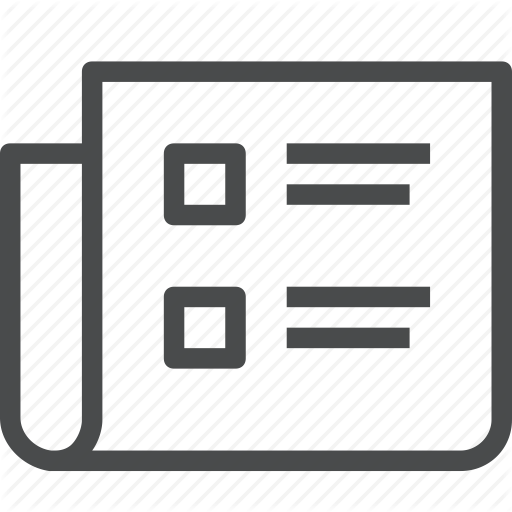
Efficient conversion of closed-channel-dominated Feshbach molecules of (NaK)-Na-23-K-40 to their absolute ground state
R. Bause, A. Kamijo, X. Y. Chen, M. Duda, A. Schindewolf, I. Bloch, X. Y. Luo
Physical Review A 104 (4), 43321 (2021).
We demonstrate the transfer of (NaK)-Na-23-K-40 molecules from a closed-channel-dominated Feshbach-molecule state to the absolute ground state. The Feshbach molecules are initially created from a gas of sodium and potassium atoms via adiabatic ramping over a Feshbach resonance at 78.3 G. The molecules are then transferred to the absolute ground state using stimulated Raman adiabatic passage with an intermediate state in the spin-orbit-coupled complex vertical bar c(3)Sigma(+), upsilon = 35, J = 1 > - vertical bar B-1 Pi, upsilon = 12, J = 1). Our measurements show that the pump transition dipole moment linearly increases with the closed-channel fraction. Thus, the pump-beam intensity can be two orders of magnitude lower than is necessary with open-channel-dominated Feshbach molecules. We also demonstrate that the phase noise of the Raman lasers can be reduced by filter cavities, significantly improving the transfer efficiency.
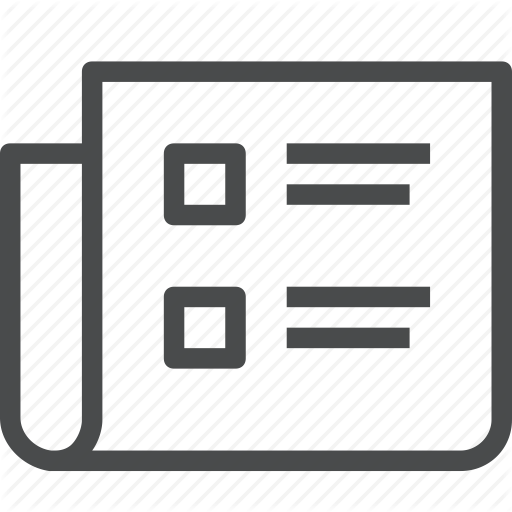
Observing non-ergodicity due to kinetic constraints in tilted Fermi-Hubbard chains
S. Scherg, T. Kohlert, P. Sala, F. Pollmann, B. H. Madhusudhana, I. Bloch, M. Aidelsburger
Nature Communications 12 (1), 4490 (2021).
The thermalization of isolated quantum many-body systems is deeply related to fundamental questions of quantum information theory. While integrable or many-body localized systems display non-ergodic behavior due to extensively many conserved quantities, recent theoretical studies have identified a rich variety of more exotic phenomena in between these two extreme limits. The tilted one-dimensional Fermi-Hubbard model, which is readily accessible in experiments with ultracold atoms, emerged as an intriguing playground to study non-ergodic behavior in a clean disorder-free system. While non-ergodic behavior was established theoretically in certain limiting cases, there is no complete understanding of the complex thermalization properties of this model. In this work, we experimentally study the relaxation of an initial charge-density wave and find a remarkably long-lived initial-state memory over a wide range of parameters. Our observations are well reproduced by numerical simulations of a clean system. Using analytical calculations we further provide a detailed microscopic understanding of this behavior, which can be attributed to emergent kinetic constraints. It was predicted that complex thermalizing behaviour can arise in many-body systems in the absence of disorder. Here, the authors observe non-ergodic dynamics in a tilted optical lattice that is distinct from previously studied regimes, and propose a microscopic mechanism that is due to emergent kinetic constrains.
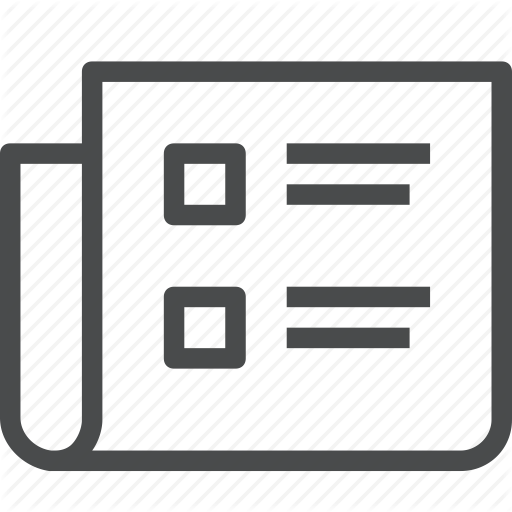
Collisions of ultracold molecules in bright and dark optical dipole traps
R. Bause, A. Schindewolf, R. H. Tao, M. Duda, X. Y. Chen, G. Quemener, T. Karman, A. Christianen, I. Bloch, X. Y. Luo
Physical Review Research 3 (3), 33013 (2021).
Understanding collisions between ultracold molecules is crucial for making stable molecular quantum gases and harnessing their rich internal degrees of freedom for quantum engineering. Transient complexes can strongly influence collisional physics, but in the ultracold regime, key aspects of their behavior have remained unknown. To explain experimentally observed loss of ground-state molecules from optical dipole traps, it was recently proposed that molecular complexes can be lost due to photoexcitation. By trapping molecules in a repulsive box potential using laser light near a narrow molecular transition, we are able to test this hypothesis with light intensities three orders of magnitude lower than what is typical in red-detuned dipole traps. This allows us to investigate light-induced collisional loss in a gas of nonreactive fermionic Na-23 K-40 molecules. Even for the lowest intensities available in our experiment, our results are consistent with universal loss, meaning unit loss probability inside the short-range interaction potential. Our findings disagree by at least two orders of magnitude with latest theoretical predictions, showing that crucial aspects of molecular collisions are not yet understood and provide a benchmark for the development of new theories.
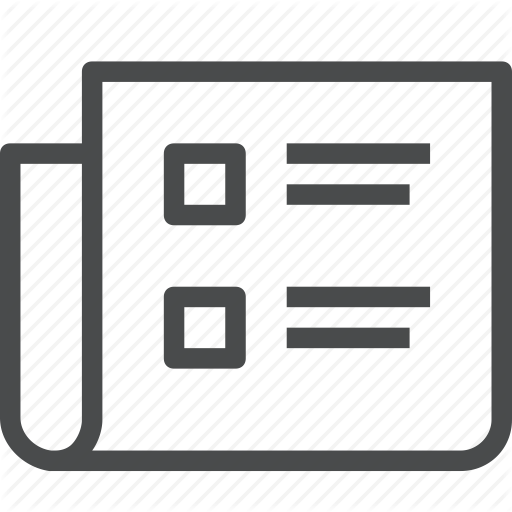
Microscopic electronic structure tomography of Rydberg macrodimers
S. Hollerith, J. Rui, A. Rubio-Abadal, K. Srakaew, D. Wei, J. Zeiher, C. Gross, I. Bloch
Physical Review Research 3 (1), 13252 (2021).
Precise control and study of molecules is challenging due to the variety of internal degrees of freedom and local coordinates that are typically not controlled in an experiment. Employing quantum gas microscopy to position and resolve the atoms in Rydberg macrodimer states solves most of these challenges and enables unique access to the molecular frame. Here, we demonstrate this approach and present photoassociation studies in which the molecular orientation relative to an applied magnetic field, the polarization of the excitation light, and the initial atomic state are fully controlled. The observed dependencies allow for an electronic structure tomography of the molecular state. We additionally observe an orientation-dependent Zeeman shift, and we reveal a significant influence on it caused by the hyperfine interaction of the macrodimer state. Finally, we demonstrate control over the electrostatic binding potential by engineering a gap between two crossing pair potentials. Our results establish macrodimers as the most sensitive tool to benchmark Rydberg interaction potentials, and they open new perspectives for improving Rydberg dressing schemes.
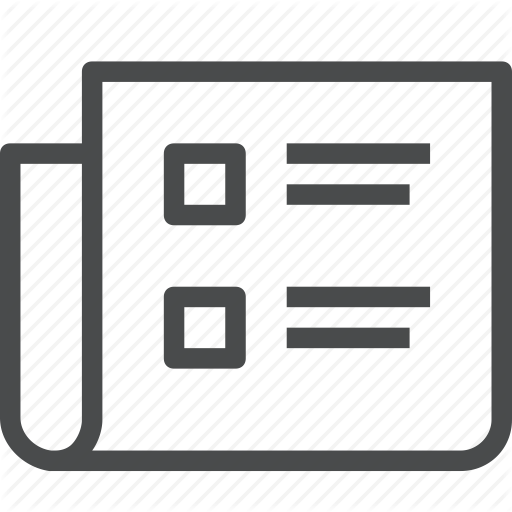
Crossed optical cavities with large mode diameters
A. Heinz, J. Trautmann, N. Santic, A. J. Park, I. Bloch, S. Blatt
Optics Letters 46 (2), 250-253 (2021).
We report on a compact, ultrahigh-vacuum compatible optical assembly to create large-scale, two-dimensional optical lattices for use in experiments with ultracold atoms. The assembly consists of an octagon-shaped spacer made from ultra-low-expansion glass, to which we optically contact four fused silica cavity mirrors, making it highly mechanically and thermally stable. The mirror surfaces are nearly plane-parallel, which allows us to create two perpendicular cavity modes with diameters similar to 1mm. Such large mode diameters are desirable to increase the optical lattice homogeneity, but lead to strong angular sensitivities of the coplanarity between the two cavity modes. We demonstrate a procedure to precisely position each mirror substrate that achieves a deviation from coplanarity of d = 1(5) mu M. Creating large optical lattices at arbitrary visible and near-infrared wavelengths requires significant power enhancements to overcome limitations in the available laser power. The cavity mirrors have a customized low-loss mirror coating that enhances the power at a set of relevant visible and near-infrared wavelengths by up to 3 orders of magnitude.. (C) 2021 Optical Society of America
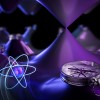
Realization of an anomalous Floquet topological system with ultracold atoms
K. Wintersperger, C. Braun, F. N. Unal, A. Eckardt, M. Di Liberto, N. Goldman, I. Bloch, M. Aidelsburger
Nature Physics 16 (10), 1058-+ (2020).
Standard topological invariants commonly used in static systems are not enough to fully capture the topological properties of Floquet systems. In a periodically driven quantum gas, chiral edge modes emerge despite all Chern numbers being equal to zero. Coherent control via periodic modulation, also known as Floquet engineering, has emerged as a powerful experimental method for the realization of novel quantum systems with exotic properties. In particular, it has been employed to study topological phenomena in a variety of different platforms. In driven systems, the topological properties of the quasienergy bands can often be determined by standard topological invariants, such as Chern numbers, which are commonly used in static systems. However, due to the periodic nature of the quasienergy spectrum, this topological description is incomplete and new invariants are required to fully capture the topological properties of these driven settings. Most prominently, there are two-dimensional anomalous Floquet systems that exhibit robust chiral edge modes, despite all Chern numbers being equal to zero. Here we realize such a system with bosonic atoms in a periodically driven honeycomb lattice and infer the complete set of topological invariants from energy gap measurements and local Hall deflections.
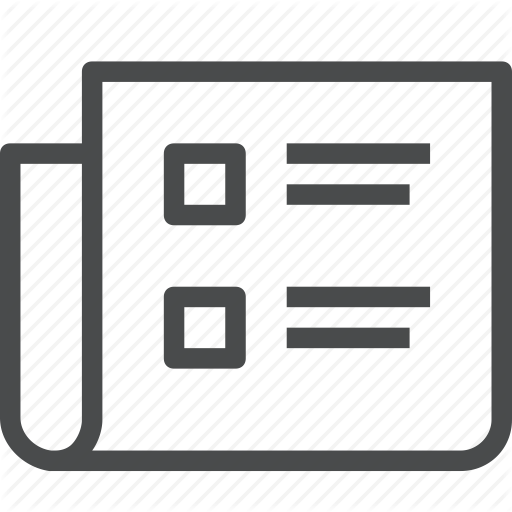
Quasiparticle Lifetime of the Repulsive Fermi Polaron
H. S. Adlong, W. E. Liu, F. Scazza, M. Zaccanti, N. D. Oppong, S. Folling, M. M. Parish, J. Levinsen
Physical Review Letters 125 (13), 133401 (2020).
We investigate the metastable repulsive branch of a mobile impurity coupled to a degenerate Fermi gas via short-range interactions. We show that the quasiparticle lifetime of this repulsive Fermi polaron can be experimentally probed by driving Rabi oscillations between weakly and strongly interacting impurity states. Using a time-dependent variational approach, we find that we can accurately model the impurity Rabi oscillations that were recently measured for repulsive Fermi polarons in both two and three dimensions. Crucially, our theoretical description does not include relaxation processes to the lower-lying attractive branch. Thus, the theory-experiment agreement demonstrates that the quasiparticle lifetime is dominated by many-body dephasing within the upper repulsive branch rather than by relaxation from the upper branch itself. Our findings shed light on recent experimental observations of persistent repulsive correlations, and have important consequences for the nature and stability of the strongly repulsive Fermi gas.
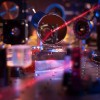
A subradiant optical mirror formed by a single structured atomic layer
J. Rui, D. V. Wei, A. Rubio-Abadal, S. Hollerith, J. Zeiher, D. M. Stamper-Kurn, C. Gross, I. Bloch
Nature 583 (7816), 369-+ (2020).
Versatile interfaces with strong and tunable light-matter interactions are essential for quantum science(1)because they enable mapping of quantum properties between light and matter(1). Recent studies(2-10)have proposed a method of controlling light-matter interactions using the rich interplay of photon-mediated dipole-dipole interactions in structured subwavelength arrays of quantum emitters. However, a key aspect of this approach-the cooperative enhancement of the light-matter coupling strength and the directional mirror reflection of the incoming light using an array of quantum emitters-has not yet been experimentally demonstrated. Here we report the direct observation of the cooperative subradiant response of a two-dimensional square array of atoms in an optical lattice. We observe a spectral narrowing of the collective atomic response well below the quantum-limited decay of individual atoms into free space. Through spatially resolved spectroscopic measurements, we show that the array acts as an efficient mirror formed by a single monolayer of a few hundred atoms. By tuning the atom density in the array and changing the ordering of the particles, we are able to control the cooperative response of the array and elucidate the effect of the interplay of spatial order and dipolar interactions on the collective properties of the ensemble. Bloch oscillations of the atoms outside the array enable us to dynamically control the reflectivity of the atomic mirror. Our work demonstrates efficient optical metamaterial engineering based on structured ensembles of atoms(4,8,9)and paves the way towards controlling many-body physics with light(5,6,11)and light-matter interfaces at the single-quantum level(7,10). A single two-dimensional array of atoms trapped in an optical lattice shows a tunable cooperative subradiant optical response, acting as a single-monolayer optical mirror with controllable reflectivity.
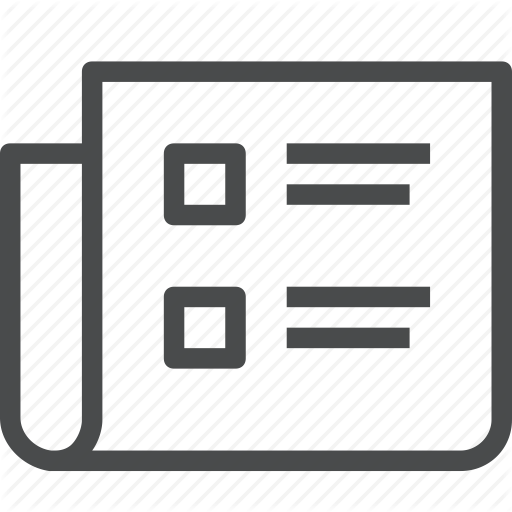
Robust Bilayer Charge Pumping for Spin- and Density-Resolved Quantum Gas Microscopy
J. Koepsell, S. Hirthe, D. Bourgund, P. Sompet, J. Vijayan, G. Salomon, C. Gross, I. Bloch
Physical Review Letters 125 (1), 10403 (2020).
Quantum gas microscopy has emerged as a powerful new way to probe quantum many-body systems at the microscopic level. However, layered or efficient spin-resolved readout methods have remained scarce as they impose strong demands on the specific atomic species and constrain the simulated lattice geometry and size. Here we present a novel high-fidelity bilayer readout, which can be used for full spin- and density-resolved quantum gas microscopy of two-dimensional systems with arbitrary geometry. Our technique makes use of an initial Stern-Gerlach splitting into adjacent layers of a highly stable vertical superlattice and subsequent charge pumping to separate the layers by 21 mu m. This separation enables independent high-resolution images of each layer. We benchmark our method by spin- and density-resolving two-dimensional Fermi-Hubbard systems. Our technique furthermore enables the access to advanced entropy engineering schemes, spectroscopic methods, or the realization of tunable bilayer systems.
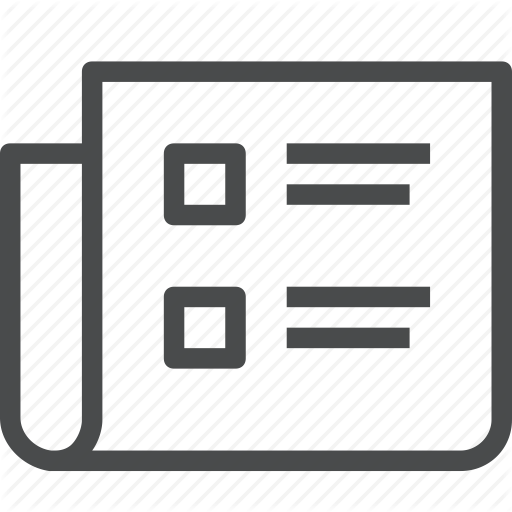
Tune-Out and Magic Wavelengths for Ground-State (NaK)-Na-23-K-40 Molecules
R. Bause, M. Li, A. Schindewolf, X. Y. Chen, M. Duda, S. Kotochigova, I. Bloch, X. Y. Luo
Physical Review Letters 125 (2), 23201 (2020).
We demonstrate a versatile, state-dependent trapping scheme for the ground and first excited rotational states of (NaK)-Na-23-K-40 molecules. Close to the rotational manifold of a narrow electronic transition, we determine tune-out frequencies where the polarizability of one state vanishes while the other remains finite, and a magic frequency where both states experience equal polarizability. The proximity of these frequencies of only 10 GHz allows for dynamic switching between different trap configurations in a single experiment, while still maintaining sufficiently low scattering rates.
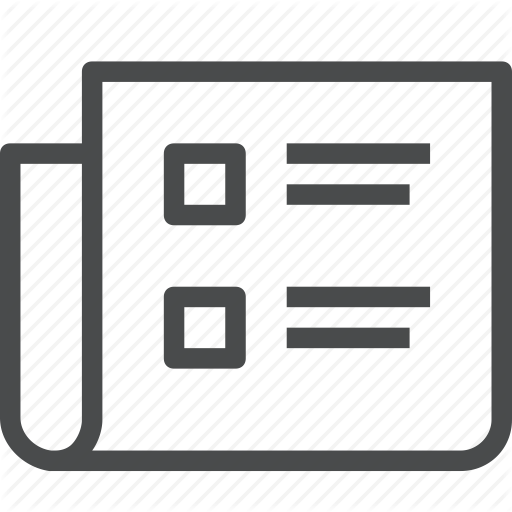
Floquet Prethermalization in a Bose-Hubbard System
A. Rubio-Abadal, M. Ippoliti, S. Hollerith, D. Wei, J. Rui, S. L. Sondhi, V. Khemani, C. Gross, I. Bloch
Physical Review X 10 (2), 21044 (2020).
"Periodic driving has emerged as a powerful tool in the quest to engineer new and exotic quantum phases. While driven many-body systems are generically expected to absorb energy indefinitely and reach an infinite-temperature state, the rate of heating can be exponentially suppressed when the drive frequency is large compared to the local energy scales of the system-leading to long-lived ""prethermal"" regimes. In this work, we experimentally study a bosonic cloud of ultracold atoms in a driven optical lattice and identify such a prethermal regime in the Bose-Hubbard model. By measuring the energy absorption of the cloud as the driving frequency is increased, we observe an exponential-in-frequency reduction of the heating rate persisting over more than 2 orders of magnitude. The tunability of the lattice potentials allows us to explore one- and two-dimensional systems in a range of different interacting regimes. Alongside the exponential decrease, the dependence of the heating rate on the frequency displays features characteristic of the phase diagram of the Bose-Hubbard model, whose understanding is additionally supported by numerical simulations in one dimension. Our results show experimental evidence of the phenomenon of Floquet prethermalization and provide insight into the characterization of heating for driven bosonic systems."
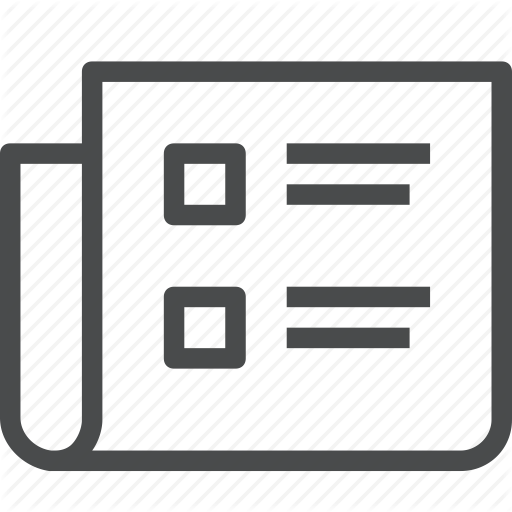
State-Dependent Optical Lattices for the Strontium Optical Qubit
A. Heinz, A. J. Park, N. Santi, J. Trautmann, S. G. Porsev, M. S. Safronova, I. Bloch, S. Blatt
Physical Review Letters 124 (20), 203201 (2020).
We demonstrate state-dependent optical lattices for the Sr optical qubit at the tune-out wavelength for its ground state. We tightly trap excited state atoms while suppressing the effect of the lattice on ground state atoms by more than 4 orders of magnitude. This highly independent control over the qubit states removes inelastic excited state collisions as the main obstacle for quantum simulation and computation schemes based on the Sr optical qubit. Our results also reveal large discrepancies in the atomic data used to calibrate the largest systematic effect of Sr optical lattice clocks.
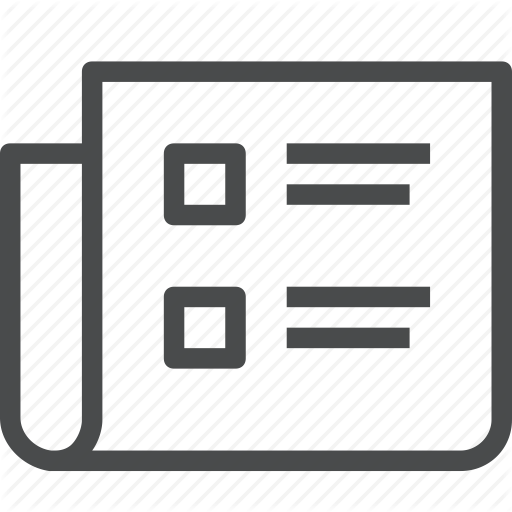
Orbital ordering of ultracold alkaline-earth atoms in optical lattices
A. Sotnikov, N. D. Oppong, Y. Zambrano, A. Cichy
Physical Review Research 2 (2), 23188 (2020).
We report on a dynamical mean-field theoretical analysis of emerging low-temperature phases in multicomponent gases of fermionic alkaline-earth(-like) atoms in state-dependent optical lattices. Using the example of Yb-173 atoms, we show that a two-orbital mixture with two nuclear spin components is a promising candidate for studies of not only magnetic but also staggered orbital ordering peculiar to certain solid-state materials. We calculate and study the phase diagram of the full Hamiltonian with parameters similar to existing experiments and reveal an antiferro-orbital phase. This long-range-ordered phase is inherently stable, and we analyze the change of local and global observables across the corresponding transition lines, paving the way for experimental observations. Furthermore, we suggest a realistic extension of the system to include and probe a Jahn-Teller source field playing one of the key roles in real crystals.
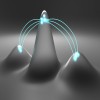
Parametric Instabilities of Interacting Bosons in Periodically Driven 1D Optical Lattices
K. Wintersperger, M. Bukov, J. Nager, S. Lellouch, E. Demler, U. Schneider, I. Bloch, N. Goldman, M. Aidelsburger
Physical Review X 10 (1), 11030 (2020).
Periodically driven quantum systems are currently explored in view of realizing novel many-body phases of matter. This approach is particularly promising in gases of ultracold atoms, where sophisticated shaking protocols can be realized and interparticle interactions are well controlled. The combination of interactions and time-periodic driving, however, often leads to uncontrollable heating and instabilities, potentially preventing practical applications of Floquet engineering in large many-body quantum systems. In this work, we experimentally identify the existence of parametric instabilities in weakly interacting Bose-Einstein condensates in strongly driven optical lattices through momentum-resolved measurements, in line with theoretical predictions. Parametric instabilities can trigger the destruction of weakly interacting Bose-Einstein condensates through the rapid growth of collective excitations, in particular in systems with weak harmonic confinement transverse to the lattice axis. Understanding the onset of parametric instabilities in driven quantum matter is crucial for determining optimal conditions for the engineering of modulation-induced many-body systems.
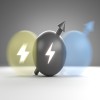
Time-resolved observation of spin-charge deconfinement in fermionic Hubbard chains
J. Vijayan, P. Sompet, G. Salomon, J. Koepsell, S. Hirthe, A. Bohrdt, F. Grusdt, I. Bloch, C. Gross
Science 367 (6474), 186-+ (2020).
Elementary particles carry several quantum numbers, such as charge and spin. However, in an ensemble of strongly interacting particles, the emerging degrees of freedom can fundamentally differ from those of the individual constituents. For example, one-dimensional systems are described by independent quasiparticles carrying either spin (spinon) or charge (holon). Here, we report on the dynamical deconfinement of spin and charge excitations in real space after the removal of a particle in Fermi-Hubbard chains of ultracold atoms. Using space- and time-resolved quantum gas microscopy, we tracked the evolution of the excitations through their signatures in spin and charge correlations. By evaluating multipoint correlators, we quantified the spatial separation of the excitations in the context of fractionalization into single spinons and holons at finite temperatures.
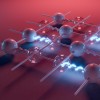
Floquet approach to Z(2) lattice gauge theories with ultracold atoms in optical lattices
C. Schweizer, F. Grusdt, M. Berngruber, L. Barbiero, E. Demler, N. Goldman, I. Bloch, M. Aidelsburger
Nature Physics 15 (11), 1168-1173 (2019).
Quantum simulation has the potential to investigate gauge theories in strongly interacting regimes, which are currently inaccessible through conventional numerical techniques. Here, we take a first step in this direction by implementing a Floquet-based method for studying Z(2) I lattice gauge theories using two-component ultracold atoms in a double-well potential. For resonant periodic driving at the on-site interaction strength and an appropriate choice of the modulation parameters, the effective Floquet Hamiltonian exhibits Z(2) I symmetry. We study the dynamics of the system for different initial states and critically contrast the observed evolution with a theoretical analysis of the full time-dependent Hamiltonian of the periodically driven lattice model. We reveal challenges that arise due to symmetry-breaking terms and outline potential pathways to overcome these limitations. Our results provide important insights for future studies of lattice gauge theories based on Floquet techniques.
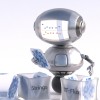
Classifying snapshots of the doped Hubbard model with machine learning
A. Bohrdt, C. S. Chiu, G. Jig, M. Q. Xu, D. Greif, M. Greiner, E. Demler, F. Grusdt, M. Knap
Nature Physics 15 (9), 921-924 (2019).
Quantum gas microscopes for ultracold atoms can provide high-resolution real-space snapshots of complex many-body systems. We implement machine learning to analyse and classify such snapshots of ultracold atoms. Specifically, we compare the data from an experimental realization of the two-dimensional Fermi-Hubbard model to two theoretical approaches: a doped quantum spin liquid state of resonating valence bond type(1,2), and the geometric string theory(3,4), describing a state with hidden spin order. This technique considers all available information without a potential bias towards one particular theory by the choice of an observable and can therefore select the theory that is more predictive in general. Up to intermediate doping values, our algorithm tends to classify experimental snapshots as geometric-string-like, as compared to the doped spin liquid. Our results demonstrate the potential for machine learning in processing the wealth of data obtained through quantum gas microscopy for new physical insights.
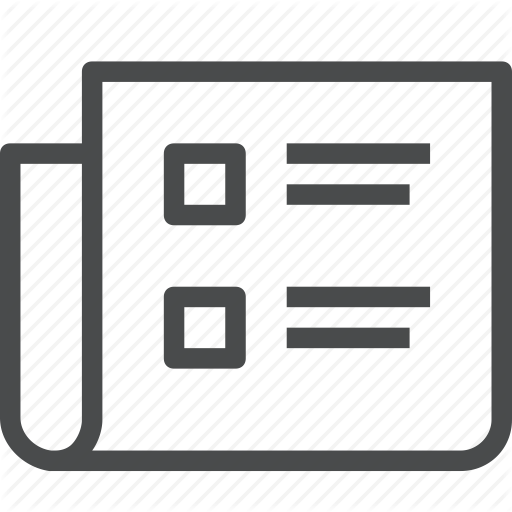
Quantum gas microscopy of Rydberg macrodimers
S. Hollerith, J. Zeiher, J. Rui, A. Rubio-Abadal, V. Walther, T. Pohl, D.M. Stamper-Kurn, I. Bloch, C. Gross
Science 364, 664-667 (2019).
A microscopic understanding of molecules is essential for many fields of natural sciences but their tiny size hinders direct optical access to their constituents. Rydberg macrodimers - bound states of two highly-excited Rydberg atoms - feature bond lengths easily exceeding optical wavelengths. Here we report on the direct microscopic observation and detailed characterization of such macrodimers in a gas of ultracold atoms in an optical lattice. The size of about 0.7 micrometers, comparable to the size of small bacteria, matches the diagonal distance of the lattice. By exciting pairs in the initial two-dimensional atom array, we resolve more than 50 vibrational resonances. Using our spatially resolved detection, we observe the macrodimers by correlated atom loss and demonstrate control of the molecular alignment by the choice of the vibrational state. Our results allow for precision testing of Rydberg interaction potentials and establish quantum gas microscopy as a powerful new tool for quantum chemistry.
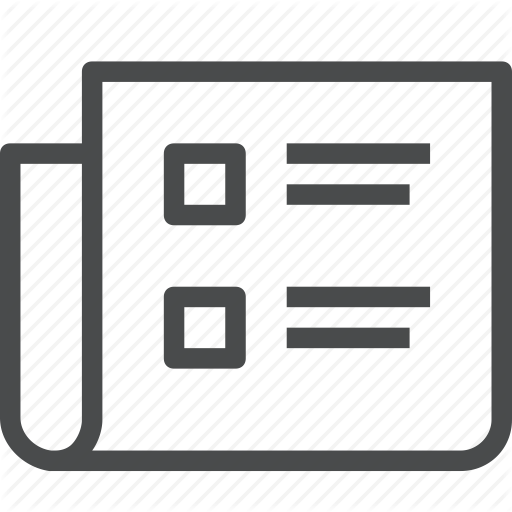
Observation of Many-Body Localization in a One-Dimensional System with a Single-Particle Mobility Edge
T. Kohlert, S. Scherg, X. Li, H. P. Luschen, S. Das Sarma, I. Bloch, M. Aidelsburger
Physical Review Letters 122 (17), 170403 (2019).
We experimentally study many-body localization (MBL) with ultracold atoms in a weak onedimensional quasiperiodic potential, which in the noninteracting limit exhibits an intermediate phase that is characterized by a mobility edge. We measure the time evolution of an initial charge density wave after a quench and analyze the corresponding relaxation exponents. We find clear signatures of MBL when the corresponding noninteracting model is deep in the localized phase. We also critically compare and contrast our results with those from a tight-binding Aubry-Andre model, which does not exhibit a singleparticle intermediate phase, in order to identify signatures of a potential many-body intermediate phase.