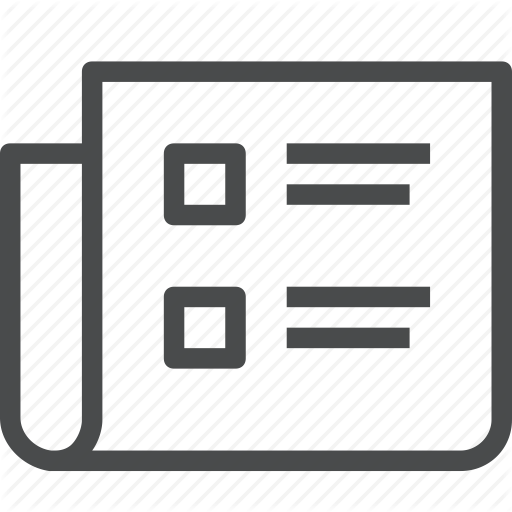
Raman sideband cooling in optical tweezer arrays for Rydberg dressing
N. Lorenz, L. Festa, L.-M. Steinert, C. Gross
Scipost Physics 10, 052 (2021).
Single neutral atoms trapped in optical tweezers and laser-coupled to Rydberg states provide a fast and flexible platform to generate configurable atomic arrays for quantum simulation. The platform is especially suited to study quantum spin systems in various geometries. However, for experiments requiring continuous trapping, inhomogeneous light shifts induced by the trapping potential and temperature broadening impose severe limitations. Here we show how Raman sideband cooling allows one to overcome those limitations, thus, preparing the stage for Rydberg dressing in tweezer arrays.
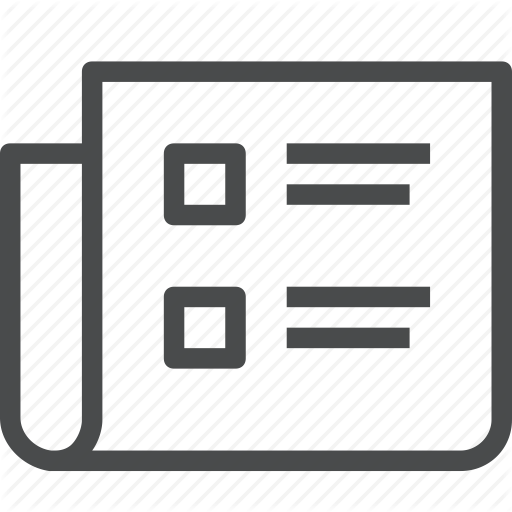
Robust Bilayer Charge Pumping for Spin- and Density-Resolved Quantum Gas Microscopy
J. Koepsell, S. Hirthe, D. Bourgund, P. Sompet, J. Vijayan, G. Salomon, C. Gross, I. Bloch
Physical Review Letters 125 (1), 010403 (2020).
Quantum gas microscopy has emerged as a powerful new way to probe quantum many-body systems at the microscopic level. However, layered or efficient spin-resolved readout methods have remained scarce as they impose strong demands on the specific atomic species and constrain the simulated lattice geometry and size. Here we present a novel high-fidelity bilayer readout, which can be used for full spin- and density-resolved quantum gas microscopy of two-dimensional systems with arbitrary geometry. Our technique makes use of an initial Stern-Gerlach splitting into adjacent layers of a highly stable vertical superlattice and subsequent charge pumping to separate the layers by 21 mu m. This separation enables independent high-resolution images of each layer. We benchmark our method by spin- and density-resolving two-dimensional Fermi-Hubbard systems. Our technique furthermore enables the access to advanced entropy engineering schemes, spectroscopic methods, or the realization of tunable bilayer systems.
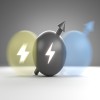
Time-resolved observation of spin-charge deconfinement in fermionic Hubbard chains
J. Vijayan, P. Sompet, G. Salomon, J. Koepsell, S. Hirthe, A. Bohrdt, F. Grusdt, I. Bloch, C. Gross
Science 10, 186-189 (2020).
Elementary particles carry several quantum numbers, such as charge and spin. However, in an ensemble of strongly interacting particles, the emerging degrees of freedom can fundamentally differ from those of the individual constituents. For example, one-dimensional systems are described by independent quasiparticles carrying either spin (spinon) or charge (holon). Here, we report on the dynamical deconfinement of spin and charge excitations in real space after the removal of a particle in Fermi-Hubbard chains of ultracold atoms. Using space- and time-resolved quantum gas microscopy, we tracked the evolution of the excitations through their signatures in spin and charge correlations. By evaluating multipoint correlators, we quantified the spatial separation of the excitations in the context of fractionalization into single spinons and holons at finite temperatures.